Introduction
Lactoferrin belongs to the transferrin family and is a non-heme iron binding glycoprotein with molecular weight of 83 kDa that contains around 690 amino acid residues (Baker and Baker, 2005). Lactoferrin is also known for its antifungal, antiviral, antimicrobial, anti-oxidant, anti-inflammatory, antiparasitic, anti-allergic and most importantly anticancer properties (Iigo et al., 2009; Parhi et al., 2012). The structure of lactoferrin consists of a single polypeptide chain which is folded into two lobes (N and C lobes) with 33 - 41% homology (González-Chávez et al., 2008). Both lobes are linked by an α-helical residue, making lactoferrin a flexible molecule. The two lobes of lactoferrin are made of α-helix and β-sheet, and each lobe can bind either Fe+2 or Fe+3 ions in synergy with the carbonate ion (CO2−) (Iafisco et al., 2011). The iron binding affinity of lactoferrin is known to be the maximum amongst transferrin family. Lactoferrin can remain bound to iron in varying pH range (Aisen and Leibman, 1972).
Lactoferrin regulates inflammatory cytokines production in a mode resembling to other anti-inflammatory cytokines by suppressing inflammation interacting with macrophages and restraining the production of inflammatory cytokines by cells (Crouch et al., 1992; Yamaguchi et al., 2001). Lactoferrin is known to suppress the production of TNF-α, IL-1β, IL-6 and IL-8 in human mononuclear cells (Haversen et al., 2002). Innate and adaptive immune response of lactoferrin makes it an important component in first line host defense mechanism against pathogens (Legrand et al, 2005; Kruzel et al., 2002).
Recent studies indicate that milk furnishes a broad range of biologically active compounds that guard neonates and adults against pathogens and illnesses, such as immunoglobulins, antibacterial peptides, antimicrobial proteins, oligosaccharides, lipids, besides many other components at low concentrations, so-called “minor” components, but with considerable potential benefits (Park and Nam, 2015).
Among those bioactive constituents in milk and dairy products, bioactive peptides (BPs) are the most studied components in this regard. BPs have been defined as specific protein fragments that have a positive impact on body function or condition and may ultimately influence health (Kitts and Weiler, 2003). BPs can be delivered to the consumers in conventional foods, dietary supplements, functional foods, or medical foods. These bioactive peptides possess very important biological activities and functionalities, including antimicrobial, antihypertensive, antioxidative, anticytotoxic, immunomodulatory, opioid, and mineral-carrying activities.
The bioactive peptides derived from a variety of dietary proteins have been reviewed by many researchers (Clare and Swaisgood, 2000; FitzGerald and Meisel, 2003; Li et al., 2004). BPs are inactive within the sequence of the parent protein and can be released in three ways: (i) through hydrolysis by digestive enzymes, (ii) through hydrolysis of proteins by proteolytic microorganisms, and (iii) through the action of proteolytic enzymes derived from microorganisms or plants (Korhonen and Pihlanto, 2007). A schematic representation of formation of bioactive peptides from major milk proteins is presented (Park and Nam, 2015).
The purpose of this study was to determine the effect of 3 types peptides (whole, above and below 10 kDa size) LH produced by alkaline protease on production of immune-modulatory activities such as IL-1β, IL-13, TNF-α and IL-6 in mouse macrophages cells named Raw264.7 cells.
Materials and Methods
Preparation of LH
Lactoferrin (Sigma Chemical, St. Louis, MO, USA) was hydrolyzed by setting it in a 2% solution to pH 8.0 with 1.0 N sodium acetate (CH3COONa), followed by heat treatment for 10 min at 85℃ (Sindayikengera and Xia, 2006; Stancius et al., 2010). The lactoferrin was cooled to 50℃, and fluid type alkaline protease (Protex 6L, Danisco, France) was added to reaction vessels as proteolytic enzymes. Protex 6L enzyme is a bacterial alkaline protease derived from a selected strain of Bacillus licheniformis and alkaline serine endopeptidase. The hydrolysis conditions are pH 9, 50℃ for 10 min and enzyme : substrate ratio is 1 : 100 followed by Protex 6L using manual. The samples were incubated using a shaking incubator (Vision Scientific Co., Ltd., Daejeon, Korea) for 2 h, then hydrolysis was stopped by heat treatment at 90℃ for 10 min. The samples was centrifuged at 12,000 g for 25 min at 4℃ and used supernatants. After cutting off by 10 kDa membrane (Vivaspin20, GE Healthcare, Sweden), the samples were centrifuged again at 8,000 g for 3 h at 4℃, and then collected both types of above and below 10 kDa LH, respectively. The samples were prepared by freezing dried and stocking at - 25℃.
Analysis of Lactoferrin hydrolysates
LH was analyzed by sodium dodecyl sulfate-polyacrylamide gel electrophoresis (SDS-PAGE) and Tricine gel (Schägger and von Jagow, 1987). The separation condition was consisted of a 10 × 8 cm, 1.5 mm thick, 10 well, 12% separating gel (tricine gel: 19%) containing acrylamide, 1.5 M tris (pH 8.8), 0.5 M tris (pH 6.8), 10% ammonium persulfate, and PAGE analysis was performed using a Mini-Protein Electrophoresis system (Bio-rad, Hercules, California, USA). LH was dissolved in sample buffer and were loaded into each well with 10 μl. The gel was stained with a solution containing 0.2% (w/v) commassie brilliant blue R-250 (Sigma Chemical, St. Louis, MO, USA) to acetic/methanol/water (1 : 1 : 5, v/v/v). Destaining solution was consisted of acetic acid/methanol/water (1 : 3 : 6, v/v/v). Molecular weights of hydrolyzed samples in the range of 12 – 250 kDa were identified using Bio-rad protein standards (Bio-rad, Hercules, California, USA).
Cell culture
The murine monocytic cell line Raw264.7 cell (ATCC, USA) was cultured in Dulbecco’s Modified Eagle Medium (DMEM) (Corning, Manassas, VA, USA) supplemented with 100 U/mL penicillin, 100 μg/mL streptomycin and 10% heat-inactivated fetal bovine serum (Atlas Biologicals, Fort Collins, Colorado, USA). The cells were cultured at 37°C in a 5% CO2 humidified incubator.
Cell viability
The mitochondrial-dependent reduction of 3-(4,5-dimethylthiazol-2-yl)-2,5-diphenyl tetrazolium bromide (MTT) to formazan was used to measure cell respiration as an indicator of cell viability (Kong, 2014). After incubation with or without 1 μg/mL lipopolysacharide (LPS) (Sigma-Aldrich, St. Louis, MO, USA) for 18 h at 37°C, cells were incubated in DMEM medium containing 0.5 mg/mL MTT for 3 h. The medium was then removed, and isopropanol was added to dissolve the formazan. After centrifugation at 5,000 g for 5 min, the supernatant of each sample was transferred to 96-well plates, and read at 570 nm in a VersaMax TM Tunable Microplate Reader (Molecular Devices Corporation, Sunnyvale, CA, USA). Negative control (without) was regarded as 100% cell viability.
Measurement of amounts of TNF-α and IL-1β
Raw264.7 cell was cultured in 24-well plate (5 × 105 cells/mL in completed medium) for 6 h at 37°C. The amount of TNF-α in the culture supernatant was analyzed after 6 h incubation in 1, 50, 100 μg/mL LH by enzyme-linked immunosorbent assay (ELISA) kit (Biolegend, San Diego, CA, USA). The quantity of IL-1β was analyzed from the culture supernatant treated with LH 50 μg/mL after 0, 3, 6, 9 h incubation by ELISA kit (Biolegend, San Diego, CA, USA). TNF-α and IL-1β were also detected by ELISA kit according to the manufacturer’s instruction. Cytokines were quantitated by the standard curves using the SOFT max curve-fitting program (Molecular Devices Co., Sunnyvale, CA, USA).
Expression of IL-6, TNF-α , IL-6 and IL-13
For detection of IL-6, TNF-α and IL-13 mRNA, reverse transcription-polymerase chain reaction (RT-PCR) was performed as follows: Cells were cultured in 12-well plate (1 × 106 cells/mL in completed medium). Three levels of LH as 1, 50, 100 μg/mL were simultaneously treated with 1 μg/mL LPS. After harvest of the cell, total RNA was isolated using Trizol reagent (Invitrogen Corporator, USA). One microgram of total RNA was converted to cDNA with 25 units/μl Oligo (dT) primer, 1 mM dNTPs, 1.75 units/μl Ribonuclease inhibitor, 2.5 units/μl M-MLV Reverse Transcriptase, M-MLV RT 5 × buffer (Promega, Madison, WI, USA). The PCR machine used was systeme-2700 made by Gene Amp PCR system 2700 (Applied Biosystems, Foster, CA, USA). The primers used are listed in Table 1.
Table 1. List of primer set for RT-PCR. ![]() |
|
GAPDH, glutaldehyde-3-phosphate dehydrogenase; TNF, Tumor necrosis factor; IL, interleukin. |
Statistical analysis
Analysis of variance was used for statistical analysis using SAS software, version 9.4 (SAS Institute Inc., USA). Duncan’s multiple range test was used to compare means, with a 5% level of significance (p < 0.05). All analyses were repeated at least 3 times and are expressed as means ± standard error (SE).
Results and Discussion
Analysis of LH
In general, since the biological activity is different depending on the molecular weight of the hydrolyzed peptides, samples are prepared by dividing into 3 types of molecular sizes (whole LH, above and below 10 kDa LH). SDS-PAGE and Tricine gel analysis revealed that almost all of LHs were hydrolyzed by the alkaline protease. A single band of 82 kDa was identified as a native lactoferrin control (Fig. 1a). Many kind of peptides derived from hydrolysates with alkaline protease had smaller molecular weight than 10 kDa (Fig. 1b). As shown in Figure 1b, the electrophoresis results of samples hydrolyzed with alkaline protease showed that the band was not clear because the various peptides with small molecular weights appear overlapping.
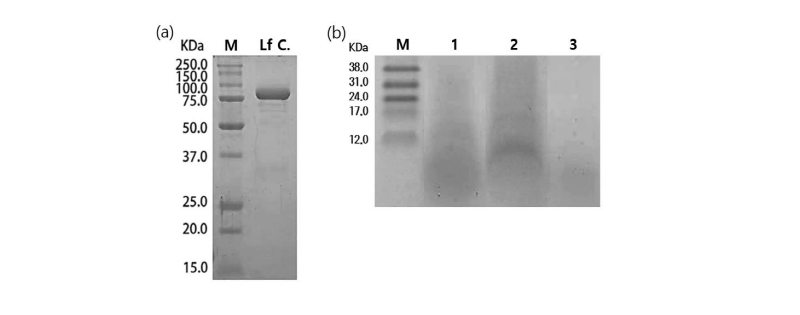
Fig. 1. Sodium dodecyl sulfate-polyacrylamide gel electrophoresis (SDS-PAGE) and tricine gel patterns of lactoferrin hydrolysis (LH) by alkaline protease. (a) M, Molecular mass of standard proteins in the range from 15 to 250 kDa; Lf C, lactoferrin control. (b) Tricine gel of LH. M, Molecular mass of standard proteins in the range from 12 to 38 kDa; Lane 1, whole LH; Lane 2, Above 10 kDa LH; Lane 3, Below 10 kDa LH.
Cell viability
The effects of the three types of LHs on cytotoxicity of the incubated Raw264.7 cells were evaluated through MTT assay (Fig. 2). No significant effects were observed on cell viability when compared the negative control with all concentrations of LHs. No differences were found between negative control and all LH treated experimental Raw264.7 cell groups, suggesting that LH did not have any cytotoxic effect on the cell culture and viability of Raw264.7 cell.
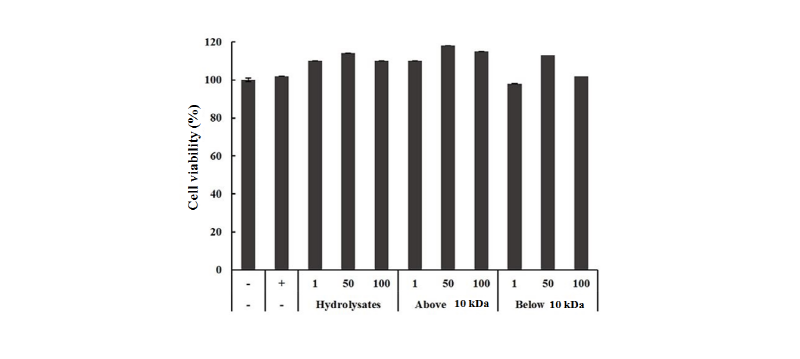
Fig. 2. Effect of lactoferrin hydrolysates on the cell viability in Raw264.7 cells for 24 hr at 37℃. Raw264.7 cells were treated with 1, 50, 100 μg/mL (whole, above and below 10 kDa) and the cells were incubated for 6 hr. LPS was treated (1 μg/mL) as a positive control. Each bar represents the average ± SE of three independent experiments. Level of significance was identified statistically compare with control using Duncan's multiple range test (p < 0.05). LPS, Lipopolysaccharide.
Cytokine production
The effect of LH on the production of pro-inflammatory cytokines including TNF-α, IL-1β, IL-6 was investigated in LPS-stimulated Raw264.7 cells. As shown in Fig. 3, one µg/mL of whole hydrolysates, above and below 10 kDa LH produced 1,500 - 2,000 ng/mL TNF-α, whereas LPS as positive control and negative control produced 2,450 ng/mL and 1,000 ng/mL, respectively. The levels of TNF-α in all LH treated groups were lower than that of LPS as positive control. However, the concentrations of TNF-α in all LH groups were decreased dose-dependently. TNF-α production was significantly different between the samples. Arai et al., (1990) also observed that the TNF-α level was decreased in their study, which is consistent with the results of our study. Haversen et al. (2002) demonstrated that Lactoferrin down-regulates the LPS-induced cytokine expression (TNF-α, IL-1β, IL-6 and IL-10) in two specific monocytic cell lines via the nuclear transcription factor kappa B (NF-κB). The production of IL-1β in Raw264.7 cells is shown in Fig. 4. The treatment of 50 µg/mL of whole LH and, above and below 10 kDa LH produced IL-1β about 20 - 28 ng/mL during at 3, 6, 9 h, while negative control had 7 ng/mL and LPS as positive control had 48 - 60 ng/mL. All 3 types of LHs had 2.5 times lower IL-1β than that of LPS positive control. IL-1β production was significantly different between the samples.
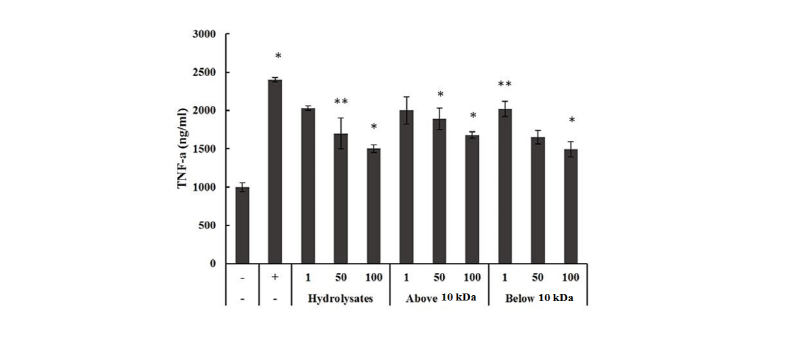
Fig. 3. Effect of lactoferrin hydrolysates on the production of TNF-α. The production of TNF-α after incubation for 6 h in Raw264.7 cells treated with lactoferrin hydrolysates 1, 50, 100 μg/mL (whole, above and below 10 kDa). LPS was treated (1 μg/mL) as a positive control. Each bar represents the average ± SE of three independent experiments. Level of significance was identified statistically compare with control using Duncan's multiple range test (*p < 0.05, **p < 0.01 ). TNF, tumor necorsis factor; LPS, Lipopolysaccharide.
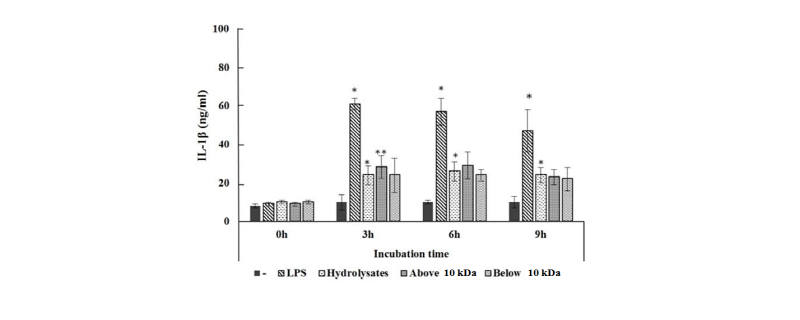
Fig. 4. Effect of by LH on the production of interleukin (IL) -1β. The production of IL-1β after incubation for 0, 3, 6, 9 h in Raw264.7 cells treated with LH 50 μg/mL (whole, above and below 10 kDa). LPS was treated (1 μg/mL) as a positive control. Each bar represents the average ± SE of three independent experiments. Level of significance was identified statistically compare with control using Duncan's multiple range test (*p < 0.05, **p < 0.01 ). LH, lactoferrin hydrolysates; IL, interleukin; LPS, lipopolysaccharide.
Fig. 5 shows that mRNA expression of cytokine in Raw264.7 cells, which were treated with all 3 types of LH at concentrations of 1, 50, 100 μg/mL for 3 h. The housekeeping gene glutaldehyde-3-phosphate dehydrogenase (GAPDH) was also affected by all LHs. The expression level of TNF-α and IL-6 were decreased dose-dependently by whole LH, above 10 kDa and below 10 kDa LH. The mRNA levels of IL-13 was slightly increased dose-dependently by whole LH and above 10 kDa, but decrease dose-dependently by below 10 kDa in the Raw264.7 cells. On the other hand, Prgomet et al., (2006) reported that the kinetics of RNA expression for TNF-α and IL-10 during culture of white blood cell and monocytes stimulated with LPS, Lactoferrin, Lactoferrincin or combinations of these macromolecular lactoferrin effected an up-regulation of the gene expression for IL-1β, IL-10, TNF-α, and IL-6 in white blood cell. These cytokines mRNA expression in white blood cell increased rapidly up to an lactoferrin concentration of 200 µg/mL, and passed into a plateau with a maximum at 1,000 µg/mL lactoferrin.
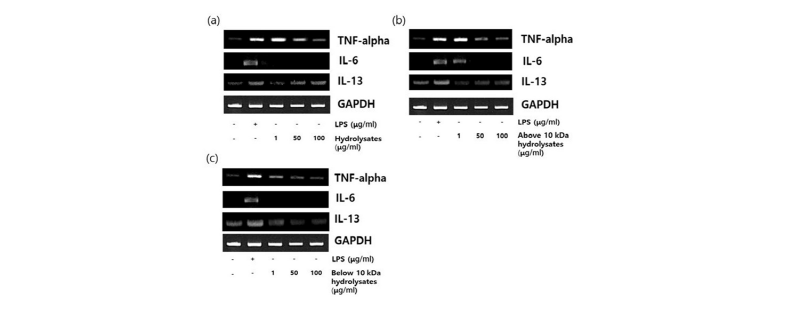
Fig. 5. Expression of TNF-α, IL-6 and IL-13 by lactoferrin hydrolysates in Raw264.7 cells . Raw264.7 cells cultured with lactoferrin hydrolysates the 1, 50, 100 μg/mL for 3 hr. LPS was treated with 1μg/mL. (a) Whole LH; (b) Above 10 kDa LH; (c) Below 10 kDa LH. TNF, Tumor necrosis factor; LH, lactoferrin hydrolysates; IL, interleukin; LPS, lipopolysaccharide; GAPDH, glutaldehyde-3-phosphate dehydrogenase.
Storcksdieck and Hurrell (2007) reported that β-casein has increased production of IL-6 as well as TNF- α by Raw264.7 cells, suggesting that β-casein may increase the production of cytokines. On the other hand, Chung et al., (2012) observed the effect of inflammatory reaction of bovine lactoferrin. The expression of the TYROBP (TYRO protein tyrosine kinase binding protein), PIYPNA (phosphatidylinositol transfer protein-alpha), IL (interleukin)-10, SLIP 9 (secretory leukocyte peptidase inhibitor), DC-STAMP (dendritic cell STAMP) and ICAM (intercellular adhesion molecular)-1 mRNA were increased by synergy effect of bovine lactoferrin and phorbol 12-myristate 13-acetate (PMA). Bovine lactoferrin and PMA had an effect of immune modulator by enhancement of TYROBO, PITPNA, SLOI, DC-STAMP, IL-10 and ICAM-1 gene transcription in U937, Mutz-3 and NK92 cells, respectively.
Conclusion
The goal of this study was to investigate on regulation of pro-inflammatory and anti-inflammatory of the bovine LH (whole, above and below 10 kDa) in a Raw264.7 cells. Our results indicated regulation of pro-inflammatory and anti-inflammatory mediators by bovine LH. These finding may be of importance enhancing the immune system when utilization of LH as a milk powder, infant food and functional food. Further studies may be need to elucidate the function of immunity mechanism by LH in vitro as well as in vivo experiments.