Introduction
As one of the most epidemic abiotic stresses, soil salinity negatively impacts on plant growth and development around the world, limiting crop productivity (Liang et al., 2014). Over 10 million hectares of farmland are abandoned every year due to soil salinity worldwide. Soil salinity affects almost half of irrigated land and approximately 20% of the farmland around the world. Land degradation due to soil salinity is an extremely crucial concern affecting the global food productivity situation (Shrivastava and Kumar, 2015). Effects of soil salinity on crop productivity are more intense in arid and semiarid areas where less rainfall, a lot of evaporation, extremely high temperature, bad water quality, and poor soil management process aggravate salinity effect on plants (Neto et al., 2006). Soil salinity significantly suppresses plant height and fructification, plant seed germination, root length, and the physiological effects of salt stress are similar to any other hyperosmotic stress like drought, cold, or freezing (Kasuga et al., 1999; Xiong et al., 2002; Munns and Tester, 2008). Soil salinity leads to membrane loss, ionic asymmetry by cause of Na+ and Cl- accumulation, increased production of reactive oxygen species like lipid peroxidation, hydroxyl radicals, hydrogen peroxide and superoxide radicals (Hasegawa et al., 2000). Furthermore, part of the biological processes and metabolic pathways related to salt stress response and tolerance are described in gene expression studies. This is consistent with the knowledge that, like all abiotic stresses, salt stress induces change in gene expression, which eventually affects the contents of proteins (Hasegawa et al., 2000; Seki et al., 2003).
Salinity stress adversely effects on plant developmental stages, especially on seed germination and delays post-germination growth (Shu et al., 2017). The difference of salt resistance may be related to the growth stage of the plant species. It is typically seen in biomass and yield reduction or in viability decrease. Salt tolerant crops have the maximum salinity level a crop tolerates without losing its productivity (Munns and Tester, 2008). Studies on the salt tolerance of various crops have shown that salt tolerance depends mainly on the genera and species, and also on the genotypes within a particular species, therefore, it is very important to identify salt-tolerant crops to improve the crop productivity in salinity land (Neto et al., 2006). As the world's population grows, the need to develop crops suitable for salt stress is increasing. Cultivation of salt tolerant crops in salinity soils helps to make the most of the salinity soils for recycling (Ngara et al., 2012).
Stress condition often results accumulation of secondary metabolites in plants (Ben Hamed et al., 2014). A secondary metabolite is not essential for the growth and life of plants, but it is required for plant-environment interaction and is generated in reaction to stresses, participating as signal molecules. As a part of plant salt stress, ionic and osmotic stress provoke the accumulation or decrease of particular secondary metabolites from plants (Zhu, 2003).
Anthocyanins are water-soluble pigments that are a sub-class of the flavonoid group discovered in the whole plant tissues. Anthocyanins are make coloration in plant for flowers and fruits. They accumulate in different plant tissues respectively under the various environmental conditions (Archetti et al., 2009; Oh et al., 2017). Anthocyanins are secondary metabolites of plants synthesized by a flavonoid biosynthetic pathway that uses phenylalanine. Anthocyanins are synthesized through a series reaction of enzyme such as chalcone isomerase, dihydroflavonol reductase, anthocyanidin synthase, flavanone 3-hydroxylase, chalcone isomerase and chalcone synthase (Tanaka et al., 2008). Anthocyanins are known to play important roles in defense from biological and abiotic stresses, providing important functions for protecting plant cells from reactive oxygen species (Chalker-Scott, 1999; Shimada et al., 2005). Simultaneously, salt stress can diminish the level of anthocyanins in salt-sensitive plant species (Liang et al., 2018). On the other hand, salt-tolerant genotypes maintain a higher accumulation of anthocyanins under salt stress compared with salt-sensitive genotypes, indicating the importance of anthocyanins in plant salinity reactions (Chutipaijit et al., 2011).
sorghum (Sorghum bicolor L.) is rather tolerant to salt stress with the 6 - 8 dSm−1 value of tolerance, which can make more biomass product than other cereal crops under salt stress (Krishnamurthy et al., 2007; Munns and Tester, 2008), thus sorghum has a great potentiality for food resource at the relatively dry and salty areas. It is also a great nutrition supply food source of minerals, calories, and proteins in developing countries (Pontieri et al., 2011). Various researches have been conducted on screening for salt tolerance of sorghum varieties (Krishnamurthy et al., 2007) and also several studies on transcriptome changes, ion accumulation, plant growth parameters, and soluble carbohydrate contents on salt stress are examined (Netondo et al., 2004; Buchanan et al., 2005; Almodares et al., 2008; Obendorf et al., 2008). Even if these studies provide useful information on the impacts of salt stress on sorghum, there needs to identify anthocyanin expression that contribute to salt tolerance mechanisms. Anthocyanin contents contribute to genotype discrimination for tolerant plants with different osmotic stress than other physiological parameters such as chlorophyll, malondialdehyde, hydrogen peroxide content (Carvalho et al., 2019). Measurement of anthocyanin expression may provide a better indication of tolerance under salt stress.
The current study aims for assessing the effect of NaCl with two sorghum genotypes based on their anthocyanin contents and gene expressions in order to determine salt tolerant genotypes. The data acquired from this study will help understanding how to deal with salt stress when the sorghum is farmed on saline soil and will provide a useful background information for breeding sorghum against salt stress.
Materials and Methods
Plants material and seed pre-germination test
Two sorghum genotypes, Sodamchal and salt tolerant Nampungchal were used in this study. It was reported that Nampungchal is more productive in reclaimed land than Sodamchal (Kang and Kwon, 2019). The seeds were obtained from Rural Development Administration (Jeonju, Korea). To evaluate tolerance of salt stress in advance, we conducted germination test with salt stress treatments. Before test, all seeds were sterilized by thiram-benomyl mixture (5 g/L) for 24 hours. Seeds were divided into two groups, first group were grown in a concentration of 200 mM NaCl and the other group were grown under normal condition. Respectively ten seeds in three petri dish for two genotypes and two treatments were germinated in a growth chamber at 25℃ (14 h light/10 h dark). After ten days, germination rate was investigated. All seeds were scored according to the degree of germination rate (shoot length), ranging from 0 to 10. Germination reducing rate is calculated by [1 - treatment sample score/control sample score] × 100.
Plants growth and stress treatment
Plants growth (including salinity imposition) was performed in a greenhouse at the Chungnam National University (CNU), Republic of Korea (36°22'06.6"N 127°21'11.3"E) from June to July 2019. Two genotypes of seeds were sown and grown until seedling stage in plastic pots; (6 cm deep with 1.5 cm diameter). When the third leaf appears, seedlings were transferred to new pots; (8 cm deep with 7 cm diameter) filled with sterilized vermiculite. A half-strength Hoagland nutrient solution (Jiang et al., 2017) was provided from the reservoir under the pots so that the nutrient solution is supplied by capillary action. Before imposing stress, plants were allowed to adapt normal nutrient solution for seven days. After adaptation to normal nutrient solution, all seedling size was unified. Stressed plants were grown with a half-strength Hoagland nutrient solutions supplemented with 50, and 150 mM NaCl for salt stress treatment and control plants were grown with normal Hoagland nutrient solutions. Leaves were harvested with three biological replicates after three, six, and nine days after stress treatment and gently washed with distilled water. Leaves were frozen with liquid nitrogen and stored at - 80℃ until use.
Quantification of anthocyanin content
The harvested sorghum samples were subjected to quantifying of anthocyanin contents. sorghum seedlings were ground into fine powder with mortar, and pestle. They were mixed with five volumes of extraction buffer (45% methanol, 5% acetic acid). The mixture was spun down for 5 minutes at 12,000 g (room temperature) and transferred supernatant to a new tube. Absorbance was measured at 530 and 657 nm through nano-MD UV-Vis Bio Spectrophotometer (Scinco, Korea) and anthocyanin contents was calculated by a following: Abs 530/g F.W. [Abs 530 - (0.25 x Abs 657)] × 5 (Nakata et al., 2013).
Quantitative RT-PCR analysis
Total RNA was extracted from six samples at Nampungchal leaves (two treatments × three biological replicates) at nine days after treatment using Trizol reagent (Sigma-Aldrich, USA), according to the manufacturer's instruction. Quality and quantity of the RNA was determined using nano-MD UV-Vis Bio Spectrophotometer (Scinco, Korea). Four genes, chalcone synthase (CHS), phenylalanine ammonia-lyase (PAL) , dihydroflavonol 4-reductase (DFR), and flavonoid 3'-hydroxylase (F3H) were subjected to quantitative real-time PCR (qRT-PCR) and accession numbers provided in Table 1 (Lo and Nicholson, 1998; Mizuno et al., 2012). The primer pairs were designed using Primer3 (v. 0.4.0) software, based on sequences available in the NCBI database and described in previous studies. It was considered the following criterion: 20 - 25 bp of primer size, GC content of 45 - 55% and melting temperature (Tm) around 60 - 62℃, product size less than 150 bp. Primers were verified by NCBI Primer-BLAST program. cDNA synthesis was processed by Compact cDNA Synthesis kit (Smart Gene, Korea) from the total RNA and cDNA samples were diluted and normalized with the house keeping gene S.vulgare SoAc1 (Huang et al., 2018). The qRT-PCR analysis was performed using CFX Connect ™ Real-Time PCR Detection System (BIO-RAD, USA) with SYBR green Q-PCR Master mix (Smart Gene, Korea). The volume of the qRT-PCR reaction was 20 µL, containing 2 µL cDNA, 10 µL 2× SYBR Green qPCR Master Mix, 2 µL of the forward and reverse primers (100 nM), and 4 µL ddH2O. PCR reactions were programed as Three-step cycle, Template denature and enzyme activation at 95℃ for 10 min, followed by 40 cycles of denature at 95℃ for 15 sec, annealing at 60℃ for 30 sec, extension 72℃ for 30 sec. Data from PCR runs were analyzed using the average of three biological replicate Ct values, normalized to the average Ct value of the housekeeping gene. Expression values were calculated using the delta dealt Ct (DDCT) method (Livak and Schmittgen, 2001).
Statistical data analysis
Data are shown as the mean of three replicates with respective SEM bars. Differences among means of each samples were tested with one-way or two-way ANOVA followed by Duncan’s multiple comparison test (p < 0.05 were considered as significant), using IBM SPSS Statistics version 24 software (IBM SPSS, Inc., Chicago, USA).
Results and Discussion
Several parameters as germination rates, anthocyanin contents, growth performance (Fig. 1), and relative gene expressions were determined in two sorghum genotypes after imposing three different NaCl concentrations including severe salt stress (150 mM NaCl), moderate salt stress (50 mM NaCl), and control with non-treatment for a period of nine days. These aspects will contribute to understanding of salt tolerance mechanisms and discriminate the different tolerance levels against osmotic stress in sorghum genotypes.
Germination test under salt stress environment
Soil salinity is one of the major abiotic stresses affecting seed germination and seedling growth around the world (Gu et al., 2018; Nedjimi and Zemmiri, 2019; Wijayasinghe et al., 2019). Seed germination has been considered good signals of plant response to salt stress. In order for the brief screening of salt stress tolerance, we first evaluated germination rate in the two sorghum genotypes under severe salt stress conditions. To study the effect of salt stress on seed germination ability, plants were evaluated during the experimental period of extreme conditions: severe salt stress treatment (200 mM NaCl) and non-treatment (control) plants. The result presented in Table 2 indicates that there was significant difference between the levels of stress treatment (p < 0.01). In the control of Sodamchal, germination score shows 6.90 ± 0.548 but during the stress imposition, it decreased to 1.20 ± 0.195. Nampungchal showed the similar tendency, of which mean germination score in control (4.13 ± 0.848) was significantly higher (p < 0.05) than stress treatment (1.36 ± 0.281). 200mM concentrations caused a significant reduction in germination rate both two genotypes. This result indicated the adverse effect of severe salt concentrations and are in coincident with those observed as regards other crops (Ahmed et al., 2006; Abazarian et al., 2011; Zanetti et al., 2019; Zhu et al., 2019). Difference between two sorghum genotypes is not significant but Sodamchal has higher seed germination reducing rate than Nampungchal. (i.e. 82.61% reduction for Sodamchal and 67.08% for Nampungchal), indirectly indicating that Nampungchal is more resistant than Sodamchal in terms of seed germination rate.
Relative anthocyanin contents under salt stress environment
Anthocyanin is a good indicator of stress responses, playing important role in protection under abiotic and biotic stress. Moreover, anthocyanins play essential functions in reproduction of plant and defense plant cells from damage by reactive oxygen species (Chalker-Scott, 1999; Shimada et al., 2005). The total anthocyanin contents in leaves of Sodamchal and Nampungchal treated with 150 mM NaCl during at three, six, and nine days after treatment (DAT) is shown in Fig. 2. At the time course of the experiment, the anthocyanin contents of Sodamchal were 0.732 ± 0.03 (3 DAT), 0.648 ± 0.041 (6 DAT) and 0.406 ± 0.024 (9 DAT) and Nampungchal were 0.598 ± 0.044 (3 DAT), 0.914 ± 0.096 (6 DAT) and 1.173 ± 0.048 (9 DAT) respectively for 150 mM NaCl treatments. Sodamchal showed a significant reduction for anthocyanin contents treated with 150 mM NaCl, as time went. However, anthocyanin contents in Nampungchal increased significantly at the same condition (p < 0.05). Furthermore, Relative anthocyanin contents of Nampungchal were about 65% higher than Sodamchal at nine days after treatment with 150 mM NaCl (p < 0.01). In Fig. 3, Effects of three levels of NaCl treatment were compared among two genotypes leaves at nine days after treatment. The relative anthocyanin contents of Sodmachal were 0.629 ± 0.03 (control), 0.458 ± 0.059 (50 mM NaCl) and 0.406 ± 0.024 (150 mM NaCl) and Nampungchal were 0.891 ± 0.173 (Control), 0.945 ± 0.090 (50 mM NaCl) and 1.049 ± 0.127 (150 mM NaCL). Significant difference of relative anthocyanin contents was observed in Sodamchal among the two stress treatment levels (control, and 150 mM NaCl treatment at nine DAT), indicating lowest level at 150 mM NaCl treatment. While, it is not significantly different in Nampungchal among stress treatments (Fig. 3). This result indicated that Nampungchal may be less salt-affected genotype than Sodamchal, as valued by anthocyanin levels under 150 mM salt stress condition at 9 DAT. Anthocyanins are secondary metabolites that can be biosynthesized in response to various osmotic stresses and play a key role in stress protection (Juszczuk et al., 2004). The production of phenyl groups on flavonoids like anthocyanins contributed to increasing salt-tolerance by binding with the toxic ions or preserving cells from ion-induced oxidative attack (Wahid and Ghazanfar, 2006). Accumulation of anthocyanin contents has been reported in some plants such as rice (Chutipaijit et al., 2011), tomato and red cabbage seedling (Eryılmaz, 2006) and transgenic potato plants (Cheng et al., 2013). In these case, higher contents of anthocyanin under stress were contribute to higher antioxidant functions and salt tolerance of plants. In this study, we pointed out that Sodamchal could be more susceptible to salt stress due to low levels of anthocyanin production under salt stress. However, Nampungchal presented higher amounts of anthocyanin contents, suggesting that Nampungchal may be less affected genotype by salt stress. These results may be consistent with the initial germination test and previous study (Kang and Kwon, 2019).
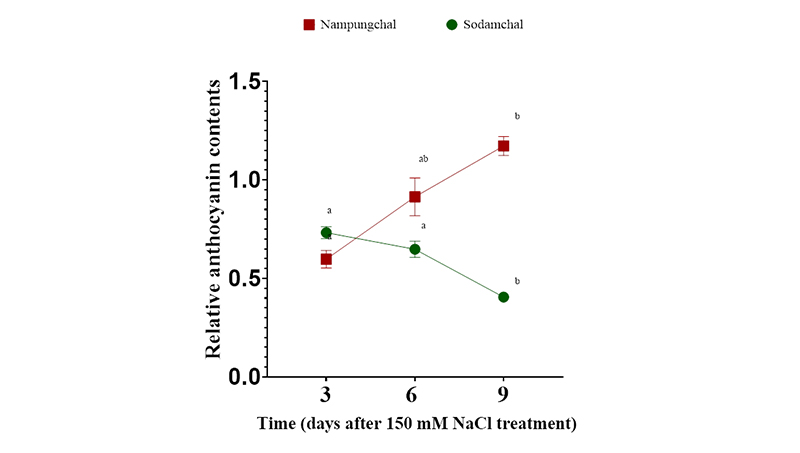
Fig. 2. Relative anthocyanin expression levels of two sorghum genotypes in leaves. Plant responses were measured with 150 mM NaCl treatment during the time course of the experiment. Values represent mean ± SEM (n = 3). a, b: Significant differences were evaluated by one-way ANOVA, followed by Duncan’s tests (p < 0.05).
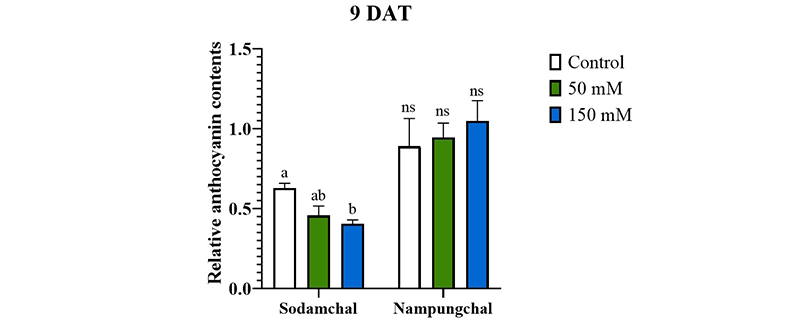
Fig. 3. Leaf anthocyanin levels of two sorghum genotypes estimated at 9 days after treatment (DAT) with severe stress treatment: 150 mM NaCl, moderate stress treatment: 50 mM NaCl, control: 0 mM NaCl Values represent mean ± SEM (n = 3). a, b: Different letters indicate significant differences among different salt stresses (ANOVA followed by Duncan’s test at p < 0.05). NS, no significant differences.
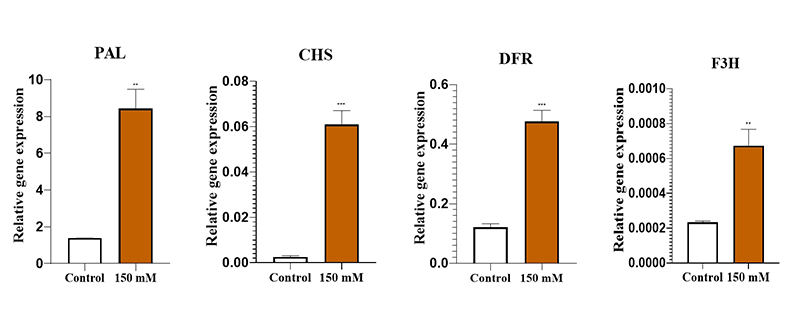
Fig. 4. Gene expression profile of Nampungchal genotypes to salt stress by quantitative RT-PCR for four genes related to anthocyanin biosynthesis. Four genes were evaluated after treatments to different salt stress intensity (0, 150 mM NaCl) at 9 days after treatment (DAT). The relative expression levels were determined after normalization with the expression reference genes, using Delta Dealt Ct (DDCT) method Values are the mean ± SEM (n = 3). Significant differences were evaluated by one-way ANOVA, followed by Duncan’s tests. *** indicates significant differences at level p < 0.001; ** indicates significant differences at level p < 0.01. PAL, flavonoid 3'-hydroxylase; CHS, chalcone synthase; DFR, phenylalanine ammonia-lyase; F3H, dihydroflavonol 4-reductase.
Genes expression profiling of anthocyanin under salt stress environment
Besides physiological markers, transcriptome expression studies have been also regarded as an important parameter for studying plant responses to various abiotic or biotic stresses. A total of four genes (CHS, DFR, F3H, PAL) possibly associated with anthocyanin biosynthesis (Table 1) were selected based on previous studies (Boss et al., 1996; Jaakola et al., 2002; Kim et al., 2007), for transcriptome expression analysis under the salt stress conditions and the profiling results of anthocyanin biosynthesis gene expression using quantitative real-time PCR are shown in Fig. 4. Based on growth performance, germination test and anthocyanin accumulation results, a tolerant genotype, Nampungchal was selected for further gene expression analysis. Changes in the expression pattern of four genes was identified in control (untreated) and salt (150 mM NaCl) treated after nine days in Nampungchal leaves. The results showed a consistent expression pattern of four genes in comparison to control. The expression levels of anthocyanin-specific pathway related genes, CHS, DFR, PAL, and F3H were significantly higher at nine days after 150 mM NaCl treatment (p < 0.01). Furthermore, a significant increase of more than ten-fold on the expression of the CHS gene in 150 mM NaCl treatments was observed (p < 0.001). Salt stress causes major changes in the expression of genes related to varied physiological processes in plants and regulatory pathways at various developmental stages (Walia et al., 2005). The difference in the expression of CHS, DFR, PAL, and F3H genes involved in anthocyanin biosynthesis pathway were an indicator of the change in total anthocyanin contents in Nampungchal genotype under salt stress. These results suggested that salt take a role of regulation in anthocyanin synthesis pathway. Increased expression patterns of CHS, DFR, PAL, and F3H genes have been reported to have a direct relationship with anthocyanin accumulation (Boss et al., 1996; Jaakola et al., 2002; Kim et al., 2007). In particular, it is reported that anthocyanin biosynthesis is associated with increased PAL activity in strawberry (Given et al., 1988), and tomato (Guo and Wang, 2010). The gene DFR which encodes anthocyanidin biosynthase is a key enzyme in the catalysis of the stereospecific dihydroflavonols reduction to leucoanthocyanidins in the purple sweet potato (Wang et al., 2013). CHS-overexpression improves abiotic stress resistance by producing more anthocyanin, enhancing photosynthetic capacity in Arabidopsis (Zhang et al., 2018). F3H enzyme was reported that it can accommodate flavanone and dihydroflavonol substrates and act at one or more levels in the anthocyanin pathway (Forkmann and Martens, 2001; Winkel-Shirley, 2001; Schlangen et al., 2009). However, the expression patterns of anthocyanin genes can be changed depending on the different developmental stages, stress intensities, and plants tissues. Research about higher expression of anthocyanin related genes in sorghum leaves is useful for screening of salt tolerant genotypes and sorghum breeding programs improving stress tolerance.
Conclusion
Salt stress is one of the serious problems in plant productivity because it delays plant growth and decreases plant development, leading the reduction of crop productivity. Searching for crops having drought tolerance are important issue in the future considering the climate changes. sorghum, rather salt tolerant crop, is a great potential as a food resource in the relatively arid and semi-arid areas. For this reason, the study of physiological and genetic responses of sorghum to salt stress may be required. In many previous studies, it has been known that, higher contents of anthocyanin under stress were contribute to higher antioxidant functions and salt tolerance of plants. In this study, the comparative analysis of two sorghum genotypes revealed difference of growth performance, anthocyanin accumulation, germination reducing rate, and gene expression, suggesting that Nampungchal is a tolerant genotype to salt stress, which it is consistent with previous study (Kang and Kwon, 2019). The Information acquired from this study will help understanding how to plants cope with salt stress when it is cultivated on saline soil, furthermore, it will be useful to estimate and use genetic resource as well as it will be a good resource for future breeding sorghum resistance to salt stress.
Authors Information
Donghyun Jeon, Chungnam National University, Master student
Solji Lee, Chungnam National University, Master student
Sehyun Choi, Chungnam National University, Master student
Sumin Seo, Chungnam National University, Undergraduate student
Changsoo Kim, Chungnam National University, Professor