Introduction
Biotechnological approaches in pigs are now more focused on biomedical sciences due to the increasing demands on the food industry, as well as its physiological contiguity to humans. Mammalian ovaries contain a large number of eggs at different stages of growth, and maturation of oocytes plays an important role in fertilization and embryo development. This process can be easily perturbed by different regulatory activities, majority of which are epigenetic modifications, apoptosis and oxidative stress (Hirao, 2011; Sharma, 2013). Considering each regulatory activity, the epigenetic modifications are known to delay oocyte maturation and provoke oocyte aging (Angrisano et al., 2010). Lipopolysaccharides (LPS) induce oxidative stress in epithelial cells, macrophages and dendritic cells (Sagar et al., 2014; Qin et al., 2015). Moreover, promoting the expression of oxidative stress-sensitive genes subsequently incites apoptosis in the cells (Raza et al., 2016). Bacterial infections in the animal reproductive system cause flaws in the proper functioning of the estrus cycle, and gram-positive bacteria secrete LPS as their main toxic compound (Hertl et al., 2010; Park et al., 2016; Zhao et al., 2017). These bacterial polysaccharides accumulate in the follicular fluid, reducing the production of estradiol from granulosa cells and causing ovarian dysfunction (Li et al., 2017). Suppression of the gonadotrophin-releasing hormone, luteal phase extension, and decrease in the conception rate, is also induced by bacterial LPS (Herath et al., 2007; Williams et al., 2008; Gilbert, 2011). Considering the advancements in the field of reproduction, assisted reproduction techniques (ART) such as in vitro maturation (IVM), fertilization (IVF) and embryo culture have gained immense attention over the past years, and are now well used in research fields (Romar et al., 2019). There is growing evidence that bacterial infections (gram negative bacteria) reduce the success rate and cause additional costs in ART (Borges et al., 2020). Hence, to gain a clearer understanding, further studies are required to interpret the effect of bacterial lipopolysaccharides in in vitro techniques. Considering all the above, the present study was undertaken to investigate the effects of LPS on the maturation of pig oocytes during IVM, and indirectly suggest cytotoxicity of germ cells caused by bacterial infection in the reproductive tract.
Materials and Methods
Collection and in vitro maturation (IVM) of pig oocytes
Ovaries were collected from prepubertal gilts at a local slaughterhouse, and transported to the laboratory. Cumulus-oocyte complexes (COCs) were aspirated from the antral follicles (3 - 6 mm in diameter), washed three times in HEPES-buffered Tyrode lactate (TL-HEPES- PVA) medium containing 0.01% (w/v) polyvinyl alcohol (PVA), followed by three washes with oocyte maturation medium (Abeydeera et al., 1998). A total of 50 COCs were transferred to 500 µL of maturation medium covered with mineral oil in a 4-well multi-dish equilibrated at 38.5℃ in an atmosphere containing 5% CO2. The oocyte maturation medium used was tissue culture medium (TCM) 199 supplemented with 0.1% PVA, 3.05 mM D-glucose, 0.91 mM sodium pyruvate, 0.57 mM cysteine, 0.5 µg·mL-1 luteinizing hormone (LH; Sigma-Aldrich, St. Louis, MO, USA), 0.5 µg·mL-1 follicle-stimulating hormone (FSH; Sigma-Aldrich, St. Louis, MO, USA), 10 ng·mL-1 epidermal growth factor (Sigma -Aldrich, St. Louis, MO, USA), 75 µg·mL-1 penicillin G, and 50 µg·mL-1 streptomycin. The oocytes were cultured in TCM199 for 44 hrs at 38.5℃, in an atmosphere comprising 5% CO2 in air, and exposed to varying concentrations of LPS (0, 1, 10, 20, and 50 µg·m-1) included in the IVM medium. All chemicals unless otherwise stated were purchased from Sigma-Aldrich.
Measurement of nitrite
Levels of nitrite (a stable product of NO) in the IVM medium were measured using the diazotization method based on the Griess reaction, as an index for NOS-derived NO production (Tunctan et al., 2005). Briefly, samples were loaded into 96-well microtiter plates, and an equal volume of Griess reagent (1% sulphanilamide and 0.1% N-1-naphthyl ethylenediamine dihydrochloride in 2.5% phosphoric acid) was added to each well. After incubation for 10 min at room temperature (RT), absorbance was measured at 550 nm with a microplate reader (Epoch, BioTek, Winooski, VT, USA). Standard curves were constructed using sodium nitrite concentrations ranging from 0.25 to 100 μM.
Fluorescence staining
For the measurement of intracellular reactive oxygen species (ROS), zona free-oocytes were incubated in TL-Hepes-PVA containing 0.5 μM carboxy-DCFDA (Invitrogen, Eugene, OR, USA) for 30 min, and washed three times in TL-Hepes-PVA. Fluorescence intensity of ROS was measured by fluorescence microscopy using the NIS-Elements Imaging Software Version 4.30 (Eclipse Ci, Nikon, Tokyo, Japan). For examining oocyte maturation, cortical granules (CGs) migration and chromosome alignment, oocytes were fixed in 2% formaldehyde for 40 min at RT, washed with PBS, permeabilized in PBS with 0.1% Triton-X 100 (PBS-TX), and blocked for 25 min in PBS-TX containing 5% normal goat serum. Oocytes were then incubated with mouse anti-β-tubulin antibody (1 : 100 dilution; Abcam, Cambridge, UK) for 40 min, washed with PBS-TX, followed by incubation with tetramethylrhodamine isothiocyanate (TRITC)-conjugated goat anti-rabbit (GAR) IgG (1 : 200 dilution), FITC-conjugated Lens culinaris agglutinin (LCA-FITC; 1 : 200 dilution) and 2.5 µg·mL-1 4’6’-diamidino-2-phenylindole (DAPI) for 40 min, and subsequently observed under fluorescence microscope (Nikon).
Statistical Analysis
Values are exhibit as the mean ± standard error of the mean (SEM). Data analyses were conducted using one-way analysis of variance (ANOVA) with SAS package 9.4 (SAS Institute Inc., Cary, NC, USA). The completely randomized design was used, and Duncan’s multiple range test was performed to compare values of individual treatments when the F-value was significant (p < 0.05).
Results and Discussion
LPS has a detrimental effect on oocytes matured in vitro
As shown in Fig. 1A, increasing LPS concentration significantly and dose-dependently decreases the maturation rate of oocytes (p < 0.05). Addition of LPS in the maturation medium during IVM resulted in incomplete metaphase II stage oocyte maturation or delayed oocyte maturation. In addition, the ratio of aberrant CG migrations and abnormal chromosome alignments was significantly higher in oocytes matured with LPS than the control group (p < 0.05, Fig. 1B). To observe the chromosome formation and spindle alignment, fluorescence staining was performed after culmination of maturation culture of oocytes. As seen in Fig. 2A-A’”, oocytes show normal CGs distribution and chromosome arrangement during maturation in the absence of LPS during IVM. However, oocytes cultured in medium containing 50 μg·mL-1 LPS showed abnormal distributions of CGs and chromosome/spindle alignment (Fig. 2B-B’”). These results indicate that LPS exposure inhibits or delays the meiotic development of oocytes, including the distribution of CGs, during IVM.
Cytokines produced by activation of the innate immune system of the host are essential for ovarian function, modulating the secretion of ovarian steroid hormones, and for proper functioning of the corpus luteum (Richards et al., 2002). Normally, these LPS interfere with the innate immune system of the host, and alter the normal biochemical reactions which negatively affect ovarian functions (Magata and Shimizu, 2017; Sun et al., 2018; Rincon et al., 2019). The results obtained in this study indicate blocking or delaying of oocyte maturation, and abnormalities in CGs distribution and chromosome/spindle alignment, in the presence of LPS during IVM (Fig. 1 and Fig. 2). Bacterial LPS interrupts the follicular phase of the ovarian cycle by interfering with endocrine events which occur before the ovulation process, inhibits secretion of LH by suppressing pulsatile GnRH secretion, and reduces the pituitary response towards GnRH (Karsch et al., 2002). High frequency of LH pulse is essential for the ovulation process. Altogether, bacterial LPS is reported to disrupt the prostaglandin mediated pathway (Karsch et al., 2002). Literatures also state that bacterial polysaccharides accumulate in the follicular fluid, thereby reducing the production of estradiol from granulosa cells, and are reported to induce cell apoptosis by interacting with the innate immune system of the host (Herath et al., 2007; Bromfield and Sheldon, 2011; Pelzer et al., 2011). Moreover, Gao et al. (2017) suggested that alterations in the GV and GVBD reduce the number of MІІ oocytes, indicating the necessity of proper endocrine functioning of the endocrine system.
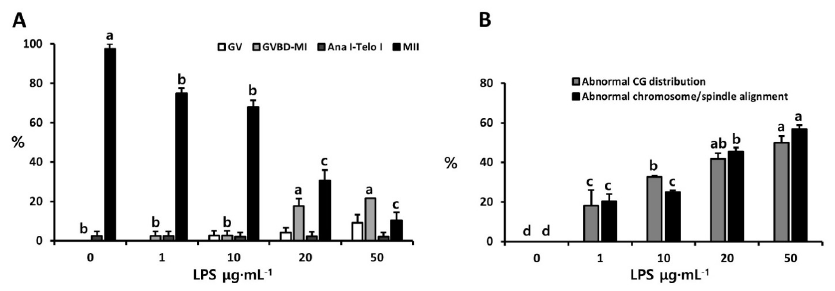
Fig. 1. Oocyte maturation in the absence or presence of lipopolysaccharides (LPS). (A) The rate of oocyte maturation after in vitro maturation (IVM) was examined and classified as germinal vesicle (GV), germinal vesicle breakdown (GVBD)-metaphase I (MI), anaphase I (Ana I)-telophase I (Telo I), and metaphase II (MII). (B) CGs migration and chromosome formation/spindle alignment were observed under fluorescence microscope. All experiments were repeated three times. Values are expressed as mean ± SEM. a-d: The different superscripts in each group of columns denote a significant difference at p < 0.05.
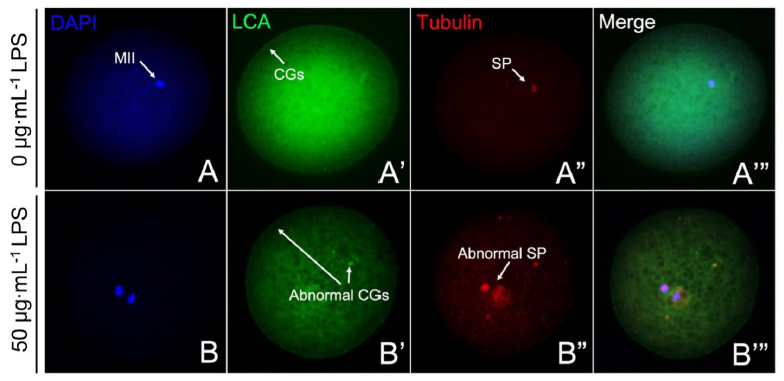
Fig. 2. Fluorescence imaging of oocytes matured in the absence (W/O) or presence of lipopolysaccharides (LPS) (50 μg·mL-1). Oocytes were stained with 4’6’-diamidino-2- phenylindole (DAPI) for DNA (blue; A & B), FITC-conjugated Lens culinaris agglutinin (LCA-FITC) for cortical granules (CGs, green; A’ & B’), and tetramethylrhodamine isothiocyanate (TRITC)- conjugated goat anti-rabbit (GAR) IgG against β-tubulin (red; A” & B”). All fluorescence colors were merged in A’” & B’”. MII, metaphase II; SP, spindle.
Generation of intracellular ROS and nitrite in oocytes matured in the presence of LPS
Compared to the control group, fluorescence intensity related to intracellular ROS was significantly increased in oocytes matured in the presence of LPS during IVM (Fig. 3A). The level of nitrite production was measured in the mature medium derived from oocytes matured in the absence or presence of LPS (Fig. 3B). We determined that the culture medium obtained from oocytes matured with LPS have higher levels of nitrite production, as compared to medium derived from oocytes matured without LPS (p < 0.05, Fig. 3B). These results indicate that LPS induces oxidative stress in the cytoplasm during oocyte maturation.
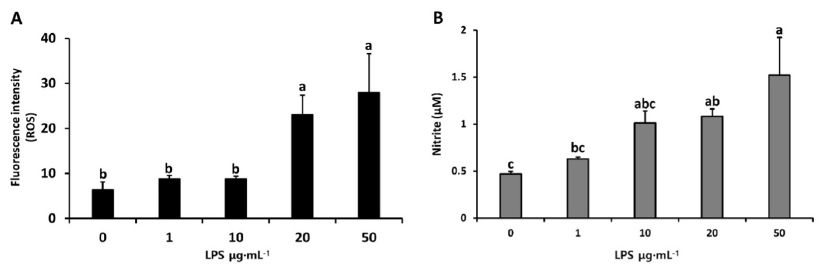
Fig. 3. Assessment of fluorescence intensity of reactive oxygen species (ROS) in oocytes matured in the absence or presence of lipopolysaccharides (LPS) (A), and nitrite level in in vitro maturation (IVM) medium obtained from oocytes matured in the absence or presence of LPS (B). All experiments were repeated three times. Values are expressed as mean ± SEM. a - c: The different superscripts in each group of columns denote a significant difference at p < 0.05.
In the present study, ROS generation was significantly and dose-dependently increased with increasing LPS concentration in the IVM medium (Fig. 3A). Optimum levels of ROS are important for oocyte maturation and fertilization, because it serves as a signaling role which is essential for regulating large number of biochemical reactions occurring in the cells (Zorov et al., 2014). Association of LPS with the immune system causes an excess secretion of ROS in the cells, which ultimately results in oxidative stress. These excess amounts of ROS negatively impact the process of oocyte maturation, follicular fluid accumulation, as well as fertilization (Siristatidis et al., 2016). Excess amounts of ROS negatively impact the cells, by causing cellular damages and promoting unwanted cell death within the living host. Altogether, excess ROS results in improper normal cell functioning (Zorov et al., 2014). Nitrite production was also significantly increased in the IVM medium derived from oocytes matured in the presence of LPS (Fig. 3B). LPS induces the production of iNOS, resulting in the production of higher amounts of NO2, which subsequently promotes apoptosis of the cells and other toxic reactions (). iNOS is important in oocyte maturation and activation. Especially, it promotes the production of cyclic guanosine monophosphate (cGMP), where the elevated cGMP levels inhibit spontaneous oocyte maturation; however, excess cGMP induces toxic reactions which reduces the proper functioning of cells (Törnell et al., 1990; Nakamura et al., 2002). In the field of ART, laboratories observing the strictest protocols are also prone to bacterial infections. Unsterilized materials, follicular fluids, semen samples, as well as the ambient air are considered to be the common pathways for microbial contamination (Borges et al., 2020). Moreover, certain infectious bacterial diseases like mastitis and metritis also impair the reproduction performances in females, as these diseases are caused by gram negative bacteria that produce LPS (Hertl et al., 2010; Sheldon et al., 2010).
Conclusion
Taken together, results from this study indicate that LPS induces oxidative stress in oocytes matured in vitro, and oocyte maturation (including meiotic development, CGs distribution and chromosome/spindle alignment) is altered in the presence of LPS during IVM of pig oocytes.
Acknowledgements
We thank Hee-Jeong Lee for experimental assistance. This research was supported by the Basic Science Research Program through the National Research Foundation of Korea (NRF) funded by the Ministry of Education (NRF-2013R1A6A3A04063769).
Authors Information
Young-Joo Yi, https://orcid.org/0000-0002-7167-5123
Adikari Arachchige Dilki Indrachapa Adikari, https://orcid.org/0000-0002-1021-3814
Seung-Tae Moon, https://orcid.org/0000-0003-1927-5250
Sang-Myeong Lee, https://orcid.org/0000-0002-3624-3392
Jung-Min Heo, https://orcid.org/0000-0002-3693-1320