Introduction
The impact of agriculture has a high influence on the world economy, resulting in farmers increasingly adopting the application of chemicals to address the escalating food demand. Among the different chemicals employed, the usage of pesticides has rampantly amplified in recent years (Gouda et al., 2018). Pesticides are defined as substances utilized to avoid, repel or destroy pests that threaten other living organisms or crops. Fipronil is one of the most applied pesticide in both the developed and developing countries (Poppenga and Oehme, 2010; Pandya, 2018; Patel and Sangeeta, 2019; Shakya et al., 2020). It is a broad-spectrum pesticide used in crop production and veterinary practice, and is categorized into the new class of pesticides called phenylpyrazole (Simon-Delso et al., 2015). Fipronil is not a volatile chemical compound, binding easily to the soil and sediment as particulate matter. Moreover, it prevails for a longer time in an aerobic environment, as compared to anaerobic or alkaline environments (Fent, 2014). It normally degrades slowly, with a half-life of 36 hrs to 7 months, and strains out slowly to the surface or in groundwater (Narahashi, 2010; Boothe, 2011; Fent, 2014).
On entering a biological body, fipronil metabolizes into fipronil sulfone, which interacts with normal cell mechanisms in the living organisms. This process is activated by cytochrome P450 3A4 (CYP3A4) and cytochrome P450 2C19 (CYO2C19) enzyme proteins (Hodgson, 2012; Singh et al., 2021). Studies on the mode of action in the insect body revealed that fipronil has the ability to inhibit the gamma-aminobutyric acid (GABA)-gated chloride channel that causes paralysis, convulsion, hyper-excitation, or death (Gant et al., 1998). Other research articles have highlighted the possible health risks associated with the pesticide in humans, like various cancers, diabetes, neurodegenerative disorders, Alzheimer’s, birth defects and reproductive disorders (Tingle et al., 2003; Souza et al., 2011; Mostafalou and Abdollahi, 2013). Studies evaluating the effects of the pesticide on mammalian reproduction reported that fipronil acts as a substance that triggers reproductive injury by reducing the sperm motility and increasing the time for achieving pregnancy (Jenardhanan et al., 2016; Bisht et al., 2017).
In male gametes, mitochondria are involved in the production of reactive oxygen species (ROS) that are important for normal cell functions. However, elevated levels in the cells due to mitochondrial dysfunction causes oxidative stress in the gametes, subsequently inducing cell apoptosis (Chen et al., 2018; Li et al., 2020). Spermatozoa are very sensitive towards oxidative stress, and exposure results in reduced sperm motility, viability and sperm-egg interactions in the host animal (Bromfield et al., 2015; Walters et al., 2018; Yang et al., 2019). Fipronil has the ability to induce the oxidative stresses that lead to various toxicities and alterations in normal metabolism (Wang et al., 2016). Damages that occur to the genital parts also results in decreased male fertility, and pesticide exposure is reported to reduce sperm motility in the cauda epididymis (Gervasi and Visconti, 2017). Previous works of literature have stated that the pesticides have the ability to downregulate the expression of genes important for the synthesis of steroid hormones (Arcondéguy et al., 2013). The current study was therefore undertaken to examine alterations of fertilization competence in boar spermatozoa exposed to fipronil.
Materials and Methods
Sperm preparation
Liquid boar semen was procured from a local artificial insemination (AI) center; samples having more than 80% sperm motility were used for the experiments. Fipronil (PESTANAL®, Cat. No. 46451, Sigma-Aldrich, St. Louis, MO, USA; Fig. 1) was dissolved with dimethyl sulfoxide (DMSO) to the required concentration. Boar spermatozoa were washed and resuspended in Beltsville thawing solution (BTS; Pursel and Johnson, 1976), followed by incubation without fipronil (or with DMSO [solvent]; controls) and or with 10 - 200 µM fipronil, at 37℃ for 30 min and 2 hrs. Unless otherwise noted, all other reagents used in this study were purchased from Sigma-Aldrich Chemical Co. LLC (St. Louis, MO, USA).
Evaluation of sperm motility
Sperm motility was examined using a computer-assisted sperm analysis system (Sperm Class AnalyzerⓇ, Microptic, Barcelona, Spain). Briefly, spermatozoa were incubated for 30 min at 37.5℃, after which 2 μL aliquot of the semen sample was placed on a pre-warmed (38℃) Leja counting slide (Leja products B.V., Nieuw-Vennep, The Netherlands), and 10 fields were examined at 37.5℃, assessing a minimum of 500 spermatozoa per sample. The proportion of total motile spermatozoa (%), progressive motile spermatozoa (%), and hyperactive spermatozoa (%) was then determined. The kinetic parameters measured for each spermatozoon included: curvilinear velocity (VCL, µm·s-1), straight-line velocity (VSL, µm·s-1), average path velocity (VAP, µm·s-1), percentage linearity (LIN, %), percentage straightness (STR, %), and wobble percentage (WOB, %).
Measurement of sperm viability
Incubated spermatozoa (1 × 108 cells·mL-1) were washed twice with phosphate-buffered saline containing 0.1% (w·v-1) polyvinyl alcohol (PBS-PVA). Following the manufacturer’s protocol, sperm viability was assayed using the LIVE/DEADⓇ Sperm Viability kit (Molecular Probes, Eugene, OR, USA), which contains the DNA dyes SYBR14 (100 nM) and propidium iodide (PI; 10 μM). The spermatozoa were stained, and images were acquired using a fluorescence microscope (Nikon Eclipse Ci microscope, Nikon Instruments Inc., Tokyo, Japan) with a camera (DS-Fi2, Nikon) and an imaging software (version 4.30, Nikon). The spermatozoa were classified and counted as viable (SYBR14) or dead (PI).
Assessment of chromatin stability
The acridine orange assay was conducted according to the Martins et al. (2007) procedure, with some modifications. Briefly, sample smears were prepared on a glass slide and allowed to air-dry, followed by overnight fixation in Carnoy’s solution (methanol : glacial acetic acid = 3 : 1). Fixed slides were air-dried again and incubated in tampon solution (80 mmol·L-1 citric acid and 15 mmol·L-1 Na2HPO4, pH 2.5) at 75℃ for 5 min. The slides were subsequently stained with acridine orange (0.2 mg·mL-1), followed by washing with water to remove the excess background stain. Coverslips were placed on the wet glass slide and observed under a fluorescence microscope equipped with an imaging software (Nikon). Spermatozoa with normal DNA content stained a green color, whereas sperm containing abnormal DNA emitted a yellow-green to red color.
Measurement of intracellular reactive oxygen species (ROS)
Spermatozoa were washed twice with 0.1% PBS-PVA, incubated with 1 µM 5-(and-6)-carboxy-2’,7’-dichlorodihydro-fluorescein diacetate (carboxy-H2DCFDA, Invitrogen, Eugene, OR, USA) at 37℃ for 10 min, and then washed twice with 0.1% PBS. Finally, the stained spermatozoa were mounted in Vectashield solution (Vector Laboratories, Burlingame, CA, USA) and observed under a fluorescence microscope equipped with an imaging software (Nikon), which measured the fluorescence intensity for ROS production in spermatozoa (Yi et al., 2021).
Statistical analysis
All experimental data are expressed as mean ± standard error of the mean (SEM), and analyzed using one-way ANOVA in GraphPad PRISM® (GraphPad software, San Diego, CA, USA). The completely randomized design was applied, and Tukey’s multiple comparison test was performed to compare values of individual treatments. Results are considered statistically significant at p-values * p < 0.05, ** p < 0.01 and *** p < 0.001.
Results and Discussion
Semen samples were exposed to varying concentrations of fipronil (10 to 200 μM) at different incubation periods (30 min and 2 hrs; Fig. 2A and 2B). As shown in Fig. 2A, a significant reduction in sperm motility was observed in the treatment groups compared to the control at 30 min incubation (83.0 - 89.0% controls vs. 78.5 - 61.5% fipronil [10 - 200 µM], p < 0.001). A similar pattern was observed in the 2 hrs incubation as well. With increasing fipronil concentration, a dose-dependent reduction in sperm motility was observed in all treatment groups (77.0 - 86.3% controls vs. 73.6 - 50.1% fipronil [10 - 200 µM], p < 0.001; Fig. 2B). Moreover, the 2 hrs incubated group showed greater reduction in sperm motility than the 30 min incubated group. Table 1 illustrates how fipronil affects the motion kinematics of sperm at different incubation periods. Compared to the controls, significant decreases were obtained in the percentages of sperm progressive motility (PR) and straightness index (STR) in spermatozoa incubated with fipronil (p < 0.01 and p < 0.001, Table 1). Moreover, as compared to the control groups, motion kinematics of curve speed (VCL), linear speed (VSL) and average path velocity (VAP) were significantly reduced in samples incubated with fipronil (p <0.05, p < 0.01, and p < 0.001, Table 1). Similar motion kinematic patterns were observed in fipronil groups incubated for 2 hrs (Table 1).
Sperm viability was assessed by staining the sperm samples with SYBR and PI stains (Fig. 3). Observations were conducted using the fluorescence microscope; the sperm population stained green color is considered as viable, whereas sperm stained red are considered as dead. Fig. 3 represents the percentages of viable and dead cell counts according to different incubation periods. As expected, significantly lower viable percentages were observed at 100 - 200 µM fipronil, as compared to controls (75.6 - 80.4% controls vs. 28.3 - 28.4% fipronil [100 - 200 µM], p < 0.01 at 30 min, Fig. 3A; 77.0 - 78.2% controls vs. 27.7% fipronil [200 µM] at 2 hrs, Fig. 3B; p < 0.05 and p <0.01). Moreover, the dead sperm percentage showed a significant and dose-dependent increase (5.8 - 6.3% controls vs. 25.0% fipronil [200 µM] at 30 min, Fig. 3C; 7.9 - 8.7% controls vs. 26.4% FPN [200 µM] at 2 hrs, Fig. 3D; p < 0.001).
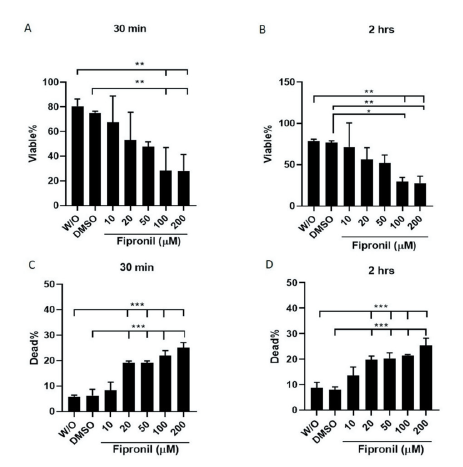
Fig. 3. Assessment of sperm viability at varying concentrations of fipronil (or controls; without [W/O] fipronil and dimethyl sulfoxide [DMSO]) and different incubation times of 30 min (A and C) and 2 hrs (B and D). Sperm that exhibit green fluorescence are considered as viable (A and B), while sperm stained with red color are considered to be dead (C and D). Values are expressed as mean ± standard error of the mean (SEM). * p < 0.05, ** p < 0.01 and *** p < 0.001
To observe the chromatin stability, semen samples were stained with acridine orange and observed under a fluorescence microscope (Fig. 4). Spermatozoa that emit a green color are considered to have normal chromatin, while sperm containing abnormal chromatin emit a yellow-green to red color. Fig. 4 shows the percentages of normal or abnormal chromatin in the sperm, and were observed to have an inversely proportional relationship. Compared to controls, the rate of normal chromatin significantly decreases with increasing fipronil concentrations after 30 min (96.1 - 96.2% controls vs. 51.6% fipronil [200 µM], p < 0.001; Fig. 4A) and 2 hrs incubation (91.6 - 93.7% controls vs. 24.1 - 28.2% fipronil [100 - 200 µM], p <0.05; Fig. 4B). Comparatively, significantly highest damaged chromatin percentage is observed at 200 μM fipronil (3.7 - 3.8% controls vs. 22.6 - 48.3% fipronil [100 - 200 µM], at 30 min, Fig. 4C; p < 0.05). In the 2 hrs incubation group, a significant decrease in normal chromatin percentages was observed at 100 and 200 μM fipronil concentrations, as compared to controls (5.0 - 5.8% controls vs. 70.2 - 73.6% fipronil [100 - 200 µM], Fig. 4D; p <0.05).
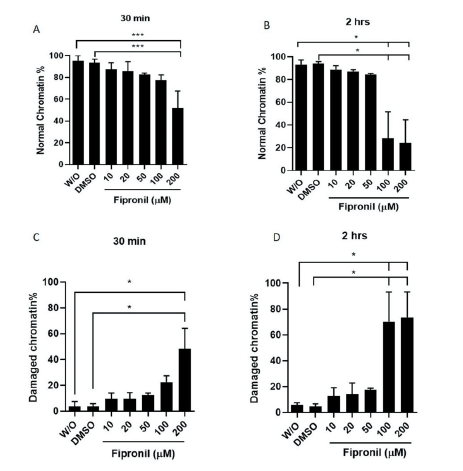
Fig. 4. Effect of fipronil on the chromatin stability of sperm at varying concentrations of fipronil (or controls; without [W/O] fipronil and dimethyl sulfoxide [DMSO]) and different incubation times of 30 min (A and C) and 2 hrs (B and D). Sperm with normal DNA content emit a green color (A and B), whereas sperm containing abnormal DNA emit a yellow-green to red color (C and D). Values are expressed as mean ± standard error of the mean (SEM). * p < 0.05 and *** p < 0.001.
Spermatozoa incubated with fipronil for 30 min show a gradual dose-dependent increase in the fluorescence intensity for ROS, at concentrations ranging from 50 to 200 μM (Fig. 5A; p < 0.05 and p < 0.01). As presented in Fig. 5B, higher fluorescence intensity was obtained in fipronil groups of 100 - 200 μM (p < 0.05 and p <0.001), as compared to the control groups. For both incubation times, highest ROS generation was obtained in spermatozoa incubated with 200 μM fipronil (Fig. 5).
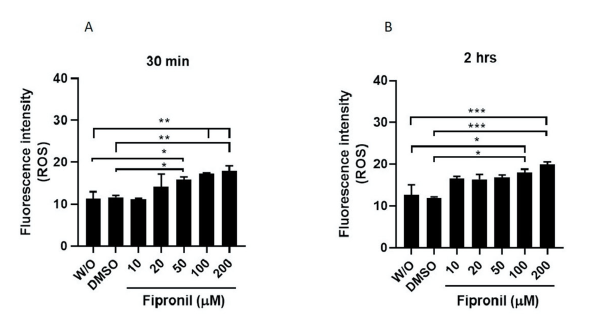
Fig. 5. Production of reactive oxygen species (ROS) in spermatozoa exposed to varying concentrations of fipronil (or controls; without [W/O] fipronil and dimethyl sulfoxide [DMSO]) and different incubation times of 30 min (A) and 2 hrs (B). Values are expressed as mean ± standard error of the mean (SEM). * p< 0.05 ** p < 0.01 and *** p < 0.001
Pesticides are one of the major environmental contaminants in the world today (Wolfe and Seiber, 1993; Mahmood et al., 2016; Rajmohan et al., 2020). Although targeted towards pests, their long-term usages increase the exposure risk for vertebrates as well (Edwards and Adams, 1970; Garcês et al., 2020). Fipronil and its metabolites are present in numerous environmental samples such as water, soil, vegetables, and several animal products (Gunasekara et al., 2007). In vertebrates, fipronil metabolizes through three pathways: oxidation-fipronil sulfone, reduction-fipronil sulfide, and hydrolysis-fipronil amide (Wang et al., 2016). Of these, fipronil sulfone is determined to be the primary active metabolite. Moreover, incubation of fipronil in the human liver microsomes (HLM) produces fipronil sulfone, and cytochrome P450 is reported to be the main enzyme involved in the oxidation of fipronil (Tan et al., 2008; ; Wang et al., 2016; Singh et al., 2021).
Fipronil controls pests by interacting with their gamma-aminobutyric acid (GABA)-gated and glutamate-gated present in the insect nervous system, by inhibiting the GABA receptors, and desensitizing or non-desensitizing the glutamate-gated chloride channels (Gant et al., 1998; Tingle et al., 2003; Kanat and Selmanoğlu, 2020). Similarly, in humans, fipronil disrupts the GABA receptors in the central nervous system, resulting in the blockage of GABA-gated chloride channels (Kanat and Selmanoğlu, 2020). This signaling system is important for regulating the hypothalamic-pituitary-gonadal (HPG) axis, and disruption of this regulating system negatively affects mammalian reproduction (Watanabe et al., 2009; Sun et al., 2014). There are two main mechanisms involved in maintaining the sperm motility: oxidative phosphorylation that is involved in the mitochondria, and the glycolysis process (Krzyzosiak et al., 1999). Apart from adenosine triphosphate (ATP) production, mitochondria are involved in ROS generation, calcium homeostasis, intrinsic apoptotic pathway, and biosynthesis of the steroid hormones (Amaral et al., 2013). Results obtained in the present study show that incubation with fipronil alters the normal functional behaviors of boar spermatozoa, including reduction in sperm motility, motion kinematics, viability, chromatin stability, and increased ROS production, thereby indicating that damages or alterations in the mitochondria potentially impairs normal sperm functions.
Fipronil has the potential to induce oxidative stress in the cells, altering the antioxidant defense systems that result in damages to lipids, proteins and DNA. Imbalance in the reactive oxygen species (ROS) causes oxidative stress that induces DNA damage and lipid peroxidation. Moreover, oxidative stress and high Ca+2 levels have the ability to extrude cytochrome c and activate the caspase cascade, resulting in apoptosis (Nichi et al., 2007; Amaral et al., 2013). All these activities are interconnected in the biological system, and interference of fipronil and its metabolites on the normal functioning of cell organelles in the sperm alters the normal sperm functions, ultimately resulting in infertility or inability to produce productive gametes. Results of the present study also corroborate the negative influences of fipronil on spermatozoa.
Conclusion
Exposure to fipronil reduced motility, motion kinematics, viability and chromatin stability in boar spermatozoa, and increased intercellular ROS production (p < 0.05). All results indicate that direct exposure of spermatozoa to fipronil leads to decreased fertilization, and interferes with male reproductive functions. In the future, it is necessary to investigate the effects of fipronil metabolites on spermatozoa.