Introduction
Chlorpyrifos is a broad-spectrum organophosphorus pesticide widely used in both residential applications (indoors, schools, restaurants, and hospitals) and agriculture for treating food crops (Rosenfeld and Feng, 2011). In general, organophosphorus pesticides rapidly degrade through exposure to sunlight, air, soil, or the soil bacterial hydrolysis process (Koshlukova and Reed, 2014). However, their effects on the biological functions of various life forms due to acute and prolonged exposure are well documented. The oxon metabolites of organophosphorus pesticides inhibit acetylcholinesterase in the nerve tissues of pests, resulting in the accumulation of acetylcholine and cholinergic hyperstimulation (Bose et al., 2021). In addition, this class of pesticides has been identified as endocrine disruptors that can interfere with the synthesis and mechanism of certain hormones, and cause carcinogenesis, neurotoxicity, hepatotoxicity, and reproductive disorders (Tanvir et al., 2016).
Early studies on the effects of chlorpyrifos on reproductive physiology have mainly focused on acute toxicity rather than the effects of chronic exposure. Recent epidemiologic studies, however, suggest that continued exposure to organophosphorus pesticide such as chlorpyrifos can impact reproductive functions. Chlorpyrifos has been shown to affect the secretion of endocrine hormones, including testosterone, follicle-stimulating hormone (FSH), and luteinizing hormone (LH). Furthermore, several studies suggest that prolonged exposure may decrease sperm count and motility, and also result in the formation of immotile and morphologically abnormal sperm (Mandal and Das, 2012; Dutta and Sahu, 2013; Ventura et al., 2016; Alaa-Eldin et al., 2017; Li et al., 2019). Moreover, chlorpyrifos and its metabolites have been known to induce oxidative stress and inhibit the activity of the enzyme P450 3A4 in testis (Buratti et al., 2003; Chen et al., 2018). Therefore, this study was conducted to examine the effects of chronic exposure to chlorpyrifos on boar spermatozoa and the male reproductive physiology.
Materials and Methods
Sperm preparation
Liquid boar semen samples were purchased from a local artificial insemination (AI) center; samples having more than 80% sperm motility were used for the experiments. Boar spermatozoa were washed and resuspended in Beltsville thawing solution (BTS; Pursel and Johnson, 1976), followed by incubation without chlorpyrifos (or with dimethyl sulfoxide [DMSO]; controls) or with 10 - 200 µM chlorpyrifos, at 37℃ for 30 min and 2 hrs. The stock solution was prepared by dissolving chlorpyrifos (PESTANAL®, #45395, Sigma-Aldrich, St. Louis, MO, USA; Fig. 1) in DMSO. Unless otherwise noted, all other reagents used in this study were purchased from Sigma-Aldrich Chemical Co. LLC (St. Louis, MO, USA).
Assessment of sperm motility
A computer-assisted sperm analysis system (Sperm Class AnalyzerⓇ, Microptic, Barcelona, Spain) was used to examine sperm motility. Boar spermatozoa were incubated for 30 min at 37.5℃, after which a 2 μL aliquot of the semen sample was placed on a pre-warmed (38℃) Leja counting slide (Leja products B.V., Nieuw-Vennep, The Netherlands), and 10 fields were examined at 37.5℃, assessing a minimum of 500 spermatozoa per sample. The proportion of total motile spermatozoa (%), progressive motile spermatozoa (%), and hyperactive spermatozoa (%) was then determined. Motion kinematic parameters such as curvilinear velocity (VCL, µm·s-1), straight-line velocity (VSL, µm·s-1), average path velocity (VAP, µm·s-1), and percentage linearity (LIN, %), percentage straightness (STR, %), and wobble percentage (WOB, %) were measured for each spermatozoon.
Evaluation of sperm viability
Sperm viability was assayed using the LIVE/DEADⓇ Sperm Viability Kit (Molecular Probes, Eugene, OR, USA), which contains the DNA dyes SYBR14 (100 nM) and propidium iodide (PI; 10 μM). First, the incubated spermatozoa (1 × 108 cells·mL-1) were washed twice with a phosphate-buffered saline solution containing 0.1% (w·v-1) polyvinyl alcohol (PBS-PVA). Thereafter the spermatozoa were stained, and images were acquired using a fluorescence microscope (Nikon Eclipse Ci microscope, Nikon Instruments Inc., Tokyo, Japan), a camera (DS-Fi2, Nikon), and imaging software (version 4.30, Nikon). The spermatozoa were classified and counted as viable (SYBR14) or dead (PI).
Measurement of acrosome integrity
Spermatozoa were fixed in a 95% ethanol solution and incubated for 30 min at 4℃. Fixed sperm were dried onto slides and stained with fluorescein isothiocyanate-labeled Pisum sativum agglutinin (FITC-PSA; 5 μg·mL-1) for 10 min (Berger, 1990). The acrosome integrity was determined using a fluorescence microscope camera and imaging software (Nikon). The images of stained samples were classified into two groups: sperm heads displaying green fluorescence were considered to have an intact acrosome while partial green fluorescence or its absence in the head was indicative of damaged or acrosome reacted spermatozoa.
Assessment of chromatin stability
Chromatin stability was evaluated by acridine orange staining as described by Martins et al. (2007) with slight modifications. Sample smears were prepared on glass slides and air-dried following overnight fixation in Carnoy’s solution (methanol:glacial acetic acid = 3 : 1) under chilling conditions. Fixed slides were again air-dried and incubated in a tampon solution (80 mM·L-1 citric acid and 15 mM·L-1 Na2HPO4, pH 2.5) at 75℃ for 5 min. Subsequently, the slides were stained with acridine orange (0.2 mg·mL-1), followed by washing to remove the excess background stain. The wet coverslips were placed on slides and observations were conducted under the fluorescence microscope equipped with a camera and imaging software (Nikon). Green fluorescence was indicative of spermatozoa containing normal DNA whereas sperm containing abnormal DNA emitted yellow-green to red fluorescence.
Measurement of intracellular reactive oxygen species (ROS)
The spermatozoa were washed twice with 0.1% PBS-PVA, incubated with 1 µM 5-(and-6)-carboxy-2’,7’-dichlorodihydro-fluorescein diacetate (carboxy-H2DCFDA, Invitrogen, Eugene, OR, USA) at 37℃ for 10 min, and then washed twice with 0.1% PBS. Finally, the stained spermatozoa were mounted in a Vectashield solution (Vector Laboratories, Burlingame, CA, USA) and observed under a fluorescence microscope equipped with imaging software (Nikon), which measured the fluorescence intensity for ROS production in the spermatozoa.
Statistical analysis
All experimental data were expressed as mean ± standard error of means (SEM) and analyzed using one-way ANOVA on the GraphPad PRISM® (GraphPad software, San Diego, CA, USA). A completely randomized design was applied, and Tukey’s multiple comparison test was performed to compare the values of individual treatments. The results were considered statistically significant at p-values *p <0.05, **p< 0.01 and ***p < 0.001.
Results and Discussion
Boar spermatozoa were incubated in the presence of chlorpyrifos (10 - 200 µM) for 30 min or 2 hrs, then assessed for sperm motility and motion kinetic parameters (Fig. 2; Table 1). Dose-dependent decrements were observed in sperm exposed to chlorpyrifos compared to controls at both incubation times (Fig. 2A and 2B). When compared to the controls after incubation for 30 min, a significant reduction in sperm motility was observed at 50 - 200 µM chlorpyrifos concentrations (81.0 - 86.9% controls vs. 76.1 - 59.3% chlorpyrifos [50 - 200 µM], p < 0.01 and p < 0.001; Fig. 2A). A similar reduction in motility was observed in the spermatozoa exposed to chlorpyrifos for 2 hrs of incubation (76.5 - 85.8% controls vs. 70.5 - 24.6% chlorpyrifos [10 - 200 µM, p < 0.01 and p < 0.001; Fig. 2B). As shown in Table 1, the percentages of sperm progressive motility (PR), straightness index (STR), curve speed (VCL), linear speed (VSL), and average path velocity (VAP) decreased in spermatozoa incubated with chlorpyrifos compared to the controls for both incubation times (p < 0.05, p < 0.01, and p < 0.001; Table 1).
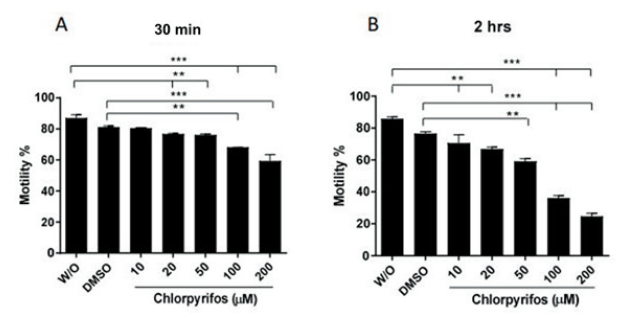
Fig.2. Evaluation of sperm motility. Boar spermatozoa were exposed to varying concentrations of chlorpyrifos (or controls; without [W/O] chlorpyrifos and dimethyl sulfoxide [DMSO]) at two different incubation times of 30 min (A) and 2 hrs (B). Values are expressed as mean ± SEM. **p < 0.01, and ***p < 0.001.
Sperm viability was evaluated after staining with SYBR14 and PI under a fluorescence microscope (Fig. 3). As shown in Fig. 3A, significantly lower viable sperm percentages were observed in the sample exposed to 200 µM chlorpyrifos (29.4%) compared to unexposed sperm (W/O: 87.9%, p < 0.05; Fig. 3A). All the chlorpyrifos-treatment groups showed significantly higher percentages of dead spermatozoa (0.2 - 6.5% controls vs. 13.0 - 39.4% chlorpyrifos [10 - 200 µM], p < 0.05, p <0.01, and p < 0.001; Fig. 3B). After 2 hrs of incubation, dose-dependent reductions of viable sperm were observed in the chlorpyrifos treatment groups (71.6 - 80.3% controls vs. 50.5 - 26.6% chlorpyrifos [10 - 200 µM], p < 0.01 and p <0.001; Fig. 3C). Similarly, higher dead sperm were observed in the sample exposed to 50 - 200 µM chlorpyrifos (14.0 - 17.7% controls vs. 45.4 - 49.7% chlorpyrifos [10 - 200 µM], p < 0.001; Fig. 3D).
Fig. 3.
Sperm viability was examined after treatment with different concentrations of chlorpyrifos (or controls; without [W/O] chlorpyrifos and dimethyl sulfoxide [DMSO]) and incubation times 30 min (A and B) and 2 hr (C and D). Spermatozoa that exhibited green fluorescence were considered viable (A and C), while sperm appearing in red color were considered to be dead (B and D). Values are expressed as mean ± SEM. *p < 0.05, **p < 0.01, and ***p <0.001.
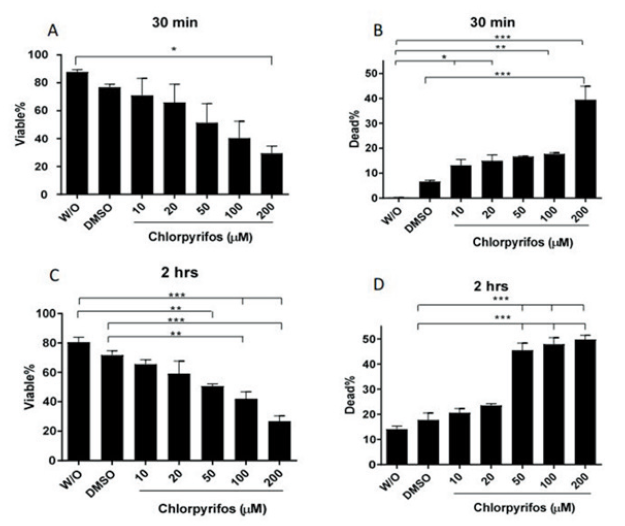
Fig. 4.
Acrosome integrity was evaluated in spermatozoa exposed to varying concentrations of chlorpyrifos (or controls; without [W/O] chlorpyrifos and dimethyl sulfoxide [DMSO]) and different incubation times of 30 min (A) and 2 hrs (B). Spermatozoa with intact acrosome emit green fluorescence, whereas spermatozoa emitting partial green fluorescence or in which the fluorescence is absent were considered damaged or acrosome reacted spermatozoa. Values are expressed as mean ± SEM. **p< 0.05 and ***p< 0.001.
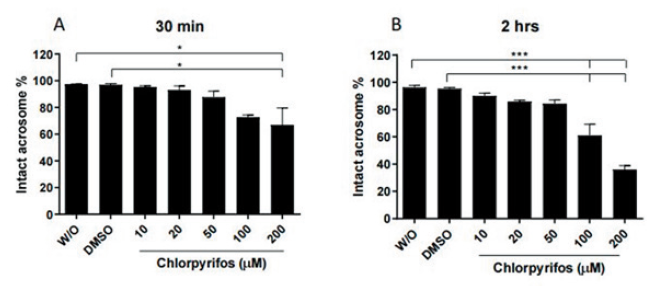
FITC-PSA staining was conducted to evaluate acrosome integrity using a fluorescence microscope (Fig. 4). As expected, the rates of intact acrosomes significantly decreased in sperm exposed to 200 µM chlorpyrifos after 30 min of incubation (96.9 - 97.2% controls vs. 66.8% chlorpyrifos [200 µM], p < 0.05; Fig. 4A), and this decrease was higher in sperm incubated with 100 - 200 µM chlorpyrifos for 2 hrs (95.3 - 96.2% controls vs. 61.0 - 35.9% chlorpyrifos [100 - 200 µM], p< 0.001; Fig. 4B).
To assess chromatin stability, sperm were stained with acridine orange (Fig. 5). Spermatozoa emitting yellow-green or red fluorescence within the sperm head were considered to have damaged chromatin, while those that emitted green fluorescence were considered to have normal chromatin (Fig. 6A - C). There was no significant difference in chromatin stability (normal or damaged chromatin) between the controls and all the chlorpyrifos-treated groups after 30 min of incubation (Fig. 5A and 5B), but the percentage of normal chromatin decreased significantly in sperm exposed to 100 - 200 µM of chlorpyrifos after 2 hrs (96.6 - 94.4% controls vs. 54.7 - 38.2% chlorpyrifos [100 - 200 µM], p < 0.001; Fig. 5C), and conversely, a significant increase in damaged DNA was observed (3.9 - 5.5% controls vs. 45.2 - 61.7% chlorpyrifos [100 - 200 µM], p < 0.01 and p < 0.001; Fig. 5D).
Fig. 5.
Chromatin stability of spermatozoa exposed to varying concentrations of chlorpyrifos (or controls; without [W/O] chlorpyrifos and dimethyl sulfoxide [DMSO]) and two different incubation times of 30 min (A and B) and 2 hrs (C and D). Normal sperm DNA emitted green fluorescence (A and C), whereas sperm containing abnormal DNA emitted yellow-green to red fluorescence (B and D). Values are expressed as mean ± SEM. The different superscripts in each group denote a significant difference at **p < 0.01 and ***p <0.001.
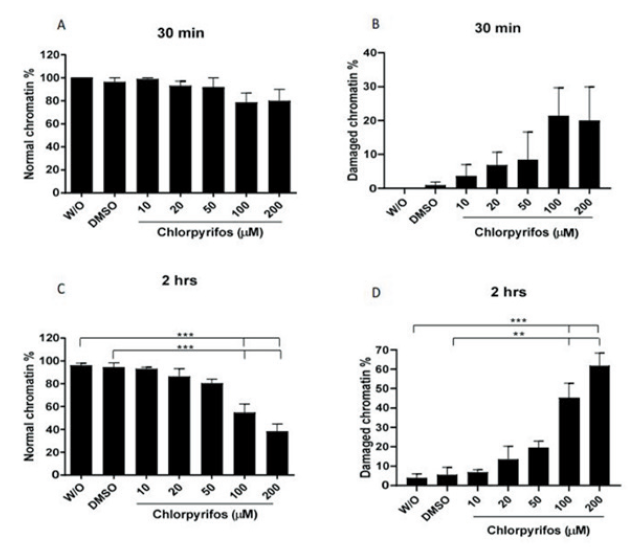
Fig. 6.
Observation of chromatin stability in spermatozoa exposed to 200 μM chlorpyrifos (or controls; without [W/O] chlorpyrifos and dimethyl sulfoxide [DMSO]) after incubation for 30 min (A - C) or 2 hrs (D - F). Green fluorescence was considered indicative of normal chromatin while abnormal chromatin emitted yellow-green to red fluorescence.
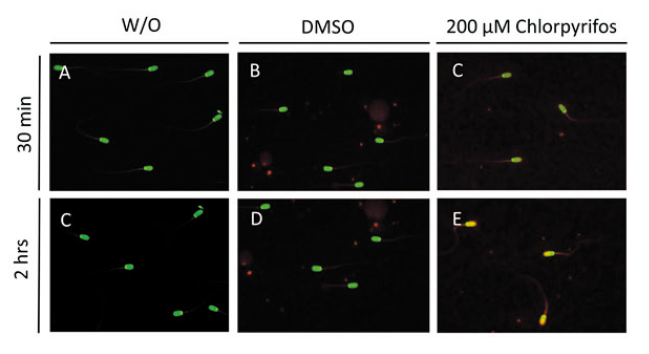
Fig. 7.
Production of reactive oxygen species (ROS) in spermatozoa exposed to varying concentrations of chlorpyrifos (or controls; without [W/O] chlorpyrifos and dimethyl sulfoxide [DMSO]) and different incubation times of 30 min (A) and 2 hrs (B). Values are expressed as mean ± SEM. **p < 0.01 and ***p <0.001.
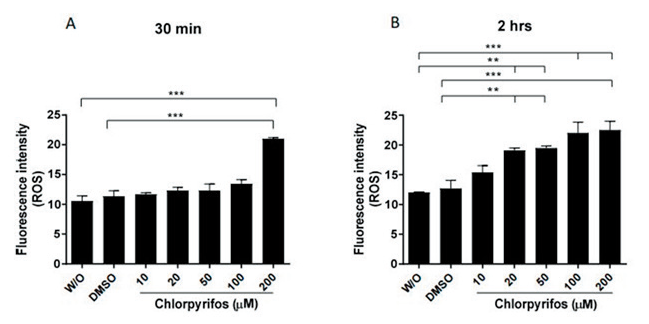
The production of ROS indicates oxidative stress in the sperm cells. As shown in Fig.7A, a higher level of ROS was observed in the sperm exposed to 200 µM chlorpyrifos than in the sperm with or without exposure to chlorpyrifos after 30 min of incubation (p < 0.001; Fig. 7A). Such increasing levels were observed more in the sperm exposed to chlorpyrifos for a longer time (fluorescence intensity [no unit]: 11.9 - 12.7 controls vs. 22.0 - 22.5 chlorpyrifos [20 - 200 µM], p < 0.01 and p <0.001).
Many studies have revealed that exposure to artificially synthesized chemicals like pesticides could threaten the health of animals as well as humans. In recent years, the rise in environmental pollution has triggered research on the negative impact of pollutants on reproductive health. (ElMazoudy et al., 2011; Dutta and Sahu, 2013; Li et al., 2019). An in vivo study by Abdel-Razik et al. (2021), revealed that oral administration of chlorpyrifos at doses of 1.9 mg·kg-1day-1, five times a week for one month in adult rats resulted in testicular deficiency with significant decline in sperm count, motility, normality, and viability. There was also significant loss in body weight. In other similar in vivo studies in experimental animals, oral exposure to chlorpyrifos resulted in a significant reduction in testicular sperm count, sperm motility, and a significant increase in the sperm malformation rate (Sai et al., 2014; Li et al., 2019; Zhang et al., 2020). In the present in vitro study also, it was observed that exposure to chlorpyrifos reduced sperm motility, motion kinematics, and viable sperm cell counts (Fig. 2 - 4).
Chlorpyrifos can induce endocrine disruption, wherein it interferes with the production of the key reproductive hormones, namely serum testosterone, LH, and FSH (Joshi et al., 2007; Alaa-Eldin et al., 2017; Abdel-Razik et al., 2021). Histological studies on the male reproductive tract after chlorpyrifos exposure have revealed the presence of defective sperm (Joshi et al., 2007; Sai et al., 2014; Adedara et al., 2018). In the current study, we observed an increase in the damage to sperm DNA with exposure to chlorpyrifos at higher concentrations and prolonged incubation time (Fig. 5). These results are similar to previous studies where chlorpyrifos and its metabolites caused chromatin alterations and DNA damage in spermatozoa (Salazar-Arredondo et al., 2008; Babazadeh and Najafi, 2017). Moreover, an increase in oxidative stress and a decrease in antioxidant activity were seen with exposure to chlorpyrifos (Abdel-Razik et al., 2021; Hassan et al., 2021) which is consistent with our current results wherein significantly higher ROS production was observed at the highest chlorpyrifos concentrations (Fig. 7).
Overall, when the pesticides were exposed to spermatozoa, oxidative stress and ROS production were increased along with cell membrane damage. In our previous results, pesticides such as fipronil significantly decreased sperm survival and degradation of fertilization competence by inhibiting the oxidative phosphorylation in mitochondria (Adikari et al., 2022). Therefore, in subsequent studies, it is necessary to investigate the effects of pesticide metabolites on the oxidative phosphorylation of spermatozoa, thereby confirming the alteration in sperm metabolism and fertilization capacity caused by toxic substances.
Conclusion
In this study, exposure of boar spermatozoa to chlorpyrifos affected their morphology and function. There was a significant reduction in motility, motion kinematics, viable cells, cells with normal intact acrosome, and chromatin stability. In addition, there was an increase in intercellular production of ROS in sperm exposed to higher concentrations of chlorpyrifos and for a longer time. Taken together, the present study indicates that direct exposure to chlorpyrifos has the potential to cause reproductive impairment in males. Further studies should be conducted to evaluate the effect of its metabolites on mammalian spermatozoa for a clear understanding of the mechanisms involved.