Introduction
Phosphorous (P) is considered to be an essential macronutrient which plays key roles in photosynthesis, sugar and energy metabolism, dividing plant cells and elongation, and transformation of information related to plant’s genetic (Rawat et al., 2020). It is also known as the master key element among all the other elements essential for the plant growth. It helps plants in surviving under hardy environmental conditions and also contributes towards disease resistance. Thus a shortfall in P availability results in reduced crop growth and production.
Agricultural soils generally contain a sizable stock of P both in inorganic and organic nature (nearly 50 to 3,000 mg·kg-1 of soil). However, low solubility along with fixation makes it very difficult for plants to uptake P from the soil solution (Zhu et al., 2018). In many tropical soils, soluble forms of P quickly convert into insoluble forms and become unavailable to plants. Consequently, about 95 - 99% of P in the soil is insoluble thus cannot be absorbed by the plants. Therefore, continuous application of P fertilizer is needed to ensure optimum plant growth. Dependency on chemical fertilizers is a matter of intensively discussed due to possible negative impacts of them on the environment and the cost involved. Under this background, adoption of new approaches that are sustainable and capable of enhancing P availability is highly important.
Soil microorganisms are known to be involved with various functions which ultimately enhance the availability of plant nutrients and thereby nutrient uptake and plant growth (Jacoby et al., 2017). In addition to chemical fertilization, P content in soil solution could be increased by the action of soil microorganisms through solubilization of insoluble forms of P. It has been identified that some microorganisms found in the environment are capable of working on insoluble forms of phosphate and making P available for plant uptake. Such microorganisms are called phosphate solubilizing microorganisms (PSMs). Through the action of them soluble and plant available orthophosphate (such as PO43--, HPO42-, and H2PO4- in soil) content in soil solution increases (Tian et al., 2021). Microbial mediated phosphate solubilization is considered as an eco-friendly and cost-effective way of soil P nutrition. As discovered by many researchers, PSMs could effectively reduce the requirement of phosphate containing fertilizers and thereby the fertilizer dependency (Li et al., 2020).
Besides phosphate solubilization, PSMs can boost plant growth through some other involvements also which include mobilizing nutrients in soils, modulating hormone levels, increasing the plants’ resistance against various diseases, enhancing the structure of the soil, detoxifying and eliminating the impact of toxic elements and breaking down the compounds. Thus the role of PSMs is recognized to be important in balancing ecological functions and thereby sustainability in producing food and feed (Rawat et al., 2020). PSMs adopt various microbial processes/ mechanisms that aid the solubilization of fixed or unavailable phosphorus (Behera et al., 2013). Production of organic acids like citric acids, oxalic acids, and gluconic acids; enzymes such as alkaline and acid phosphatases, phytases; and siderophore like ion chelators are some of them. The content presented in this review focused on an overview of PSMs and different mechanisms adopted by PSMs to solubilize insoluble phosphate.
Phosphate solubilizing microorganisms
Soil microorganisms are involved in multiple ecosystem functions, including litter decomposition, transformation of insoluble nutrients into soluble forms, primary production and the regulation of greenhouse emissions (Wagg et al., 2014). Evidence of their involvement in phosphate solubilization is dates back to 1903. By way of solubilization and mineralization, PSMs are involved in soil P cycle which ultimately facilitates availability and plant uptake of P.
PSMs are naturally found in a wide range of environmental conditions and their abundance depends on the nature of soil as well. Physical, chemical and biological properties of soil and its P content determine the diversity and the abundance of PSMs in soil. However, the degree of success of phosphate solubilizing varies with the zone of the origin of PSMs. In this connection, microorganisms isolated from the rhizoplane are reported to exhibit the highest capacity followed by rhizosphere with intermediate capacity and the least with non-rhizosphere. Compared to the non-rhizosphere soil, a considerably higher number of PSMs is commonly found in the rhizosphere soil. As reported by Kishore et al. (2015), not only bacterial and fungal species, some species of blue green algae, actinomycetes, and protozoa are known to have the ability to phosphate solubilization and mineralization (Table 1). However, by contrast, the efficacy of phosphate solubilization in bacteria is higher than the other organisms. The relative abundance of phosphate solubilizing bacteria (PSB) in soil could vary between 1 - 50%, while the contribution from phosphate solubilizing fungi (PSF) could be as low as 0.1 - 0.5% (Behera et al., 2013).
Among the different PSMs, bacterial strains coming under genera Burkholderia, Bacillus, Pseudomonas, Enterobacter, Rhizobium and Arthrobacter, and fungal strains coming under genera Aspergillus, and Penicillium have been identified as the most effective phosphate solubilizes.
Besides facilitating P availability, plant growth promotion is known to be another important contribution of PSMs. Growth enhancement even under stressed conditions is particularly important. As presented in Table 2, growth promoting effects of PSMs have been investigated using the plants grown in pots as well as under field conditions. As revealed by them, PSMs are proved to have stimulating effects on (a) nitrogen fixation; (b) accessibility of trace elements such as iron, zinc; and (c) production of plant growth promoting substances like gibberellins, auxins and cytokinins. Furthermore, they are capable of facilitating and enhancing the production of siderophores, excretion of some vitamins, synthesis of antibiotics and enzymes. As indicated by many researchers, phosphorus nutrition in natural and as well as in agricultural ecosystems is enriched by associations exist among plat roots and PSMs. The existing literature is rich with the reports on isolation, identification and characterization of PSMs with the potential use as biofertilizers.
Mechanisms of phosphate solubilization
A number of research findings elaborating the possible mechanisms which are involved in solubilizing the insoluble forms of P is available in the literature. Dissolution and precipitation, sorption and desorption, and mineralization and immobilization are identified as the major processes in this regard (Sims and Pierzynski, 2005). Generally plants could uptake P only if the dissolution and mineralization rate of P exceeds immobilization. As depicted in Fig. 1, process of phosphate solubilization may include; (a) secreting and making available of chelating or mineral dissolving compounds such as organic acid anions, siderophores, H+, OH-, and CO2, (b) making available of extracellular enzymes like acid and alkaline phosphatases, phytases and (c) releasing P though the breaking down of organic matter (Rawat et al., 2020).
Fig. 1. >Diagrammatic presentation of the mechanisms of PSMs mediated phosphate solubilization. The plate in the figure depicts phosphate solubilizing fungi (Aspergillus awamori bxq33110), which was isolated by the author and sequences were deposited in the NCBI genebank under accession number KF836501.
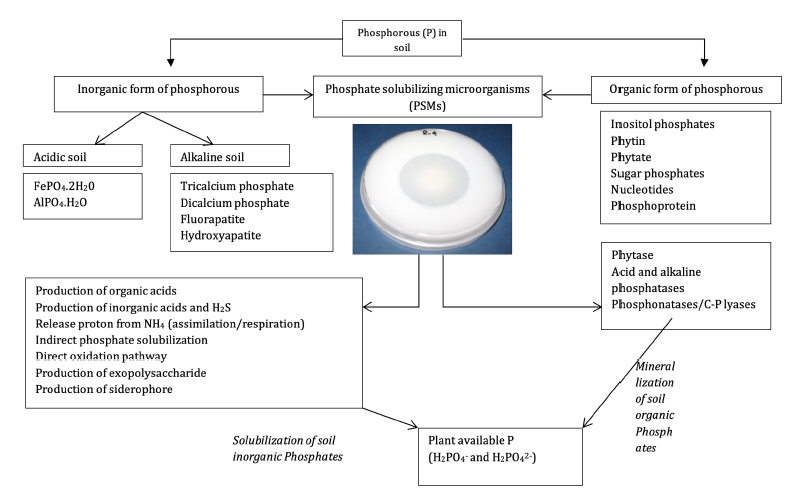
Inorganic phosphate solubilization
With regard to the solubilization of inorganic phosphate sources such as Calcium, Aluminum and Iron phosphates, involvement of several substances such as organic acids and inorganic acids, H2S, siderophores and exopolysaccharides (EPS) and actions such as release of proton from NH4+ assimilation/respiration, indirect dissolution and direct oxidation have been discussed (Kishore et al., 2015).
Organic acid production
As reported by several authors P solubilization takes place primarily with the involvement of several organic acids synthesized and secreted by PSMs (Sharma et al., 2013). In this context, use of organic anions instead of organic acids would be the more appropriate term. Many organic acid anions generally have low acid dissociation constants (pKa). Therefore, these low molecular weight substances exist in the cytosol of cells and in soil as dissociated anions across a wide range of pH. Though secretion of organic anion is often found to be associated with medium acidification, such anions are not expected to acidify the medium as they are already in their dissociated forms. This happens as a result of releasing H+ in compensating the reduction of net negative charges that could cause medium acidification (Jones and Oburger, 2011).
The organic acids could involve with phosphate solubilization through 1) acidifying rhizosphere, 2) facilitating the chelation of cations responsible of precipitation of phosphate, 3) making available of metal ions which are associated with insoluble Ca, Al, and Fe phosphates compounds, 4) competing with P for occupying the adsorption sites on the soil (Kishore et al., 2015). In order to acidify the medium, phosphate solubilizing bacteria, fungi, and actinomycetes produce various low molecular weight organic acids which could convert insoluble inorganic phosphate to soluble orthophosphate forms (PO43-, HPO42-, and H2PO4-). The production of organic acids is basically done by oxidative respiration and fermentation of organic carbon sources or sometimes through the direct oxidation pathway which acts on the outer face of the cytoplasmic membrane (Shrivastava et al., 2018). The organic acids could then dissolve the phosphates by acidifying the soil solution, which ultimately facilitate anion exchange of PO42- by acid anions or they could ensure the release of phosphate by chelating heavy metal ions such as Ca, Al and Fe with them. The monovalent anion phosphate (H2PO4-) is found to be the key soluble form of inorganic phosphate, which often exists at low pH ranges. However, once the medium pH increases, the divalent (HPO4-2) and trivalent (PO43-) forms of inorganic P are formed. Therefore, the production of organic acids by PSMs and subsequent release into the soil solution could lead acidifying the cells and their surrounding environment which eventually discharge P ions from the phosphate containing minerals as they substitute H+ for the cations bound to phosphates (Sharma et al., 2013).
The nature and the amount of organic acids synthesized by PSMs depend upon the type of organism who is involved with the process. The organic acids synthesized and discharged by PSMs include citric acid, lactic acid, gluconic acid, 2-ketogluconic acid, oxalic acid, tartaric acid, succinic, malonic, glutaric, malic and acetic acid (Table 3). Phosphate solubilizing efficiency is depended upon the nature and strength of the organic acid (Behera et al., 2013) and gluconic acid and 2-ketogluconic acid are found to be the dominant agents of inorganic phosphate solubilization (Song et al., 2008). The nutrient status of a soil is therefore influenced by the capacity of the organisms to secrete organic acids and phosphate solubilization. According to Magallon-Servin et al. (2020), who studied the mineralogy of the soil and the nature of fungi which influence the status of organic acid profile in the soil reported that phosphate solubilization efficiency is not necessarily linked to the nature or the content of organic acid produced by the microorganism. Therefore microorganisms which release fewer organic acids are more efficient in phosphate solubilization compared to those which release more organic acids with high acidity constants/coefficients (Li et al., 2016). Usually, fungi are considered to be more efficient in phosphate solubilization than bacteria which might be due to the higher acidity constants of organic acids produced by bacteria compared to the fungi (Silgado et al., 2020). In this context, tri and di-carboxylic acids are found to be more efficient than mono basic and aromatic acids. Furthermore by contrast, phenolic, citric and fumaric acids are lesser effective in phosphate solubilization than aliphatic acids (Mahidi et al., 2011). This further explains the lower efficiencies of phosphate solubilization exhibited by monocarboxylic acids (acetic, formic, lactic, and gluconic acids) compared to di- and tri-carboxylic acids (oxalic, malic, and citric acids), which have higher acidity coefficients (Jiang et al., 2020). Paper chromatography, thin layer chromatography and high performance liquid chromatography have been employed in detecting the organic acids during the process of phosphate solubilization which has also been confirmed some enzymatic methods as well. However, as reported by Khan et al. (2009), definite correlation between the proportions of acids produced by PSMs and amounts of phosphate solubilized has yet been reported.
Use of genetic markers to characterize phosphate solubilizing capacity of the PSMs has not yet been widely reported. However, efforts have been made to detect PSMs activity using PCR-based gene specifc methods (Alaylar et al., 2019). PSMs certainly contain numerous phosphate solubilizing genes. Early reports are available on the discovery of phosphate encoding gene (Pyrroloquinoline quinone/PQQ synthetase gene) in Erwinia herbicola, a species known to have the capacity to solubilize phosphates (Goldstein and Liu, 1987). Metabolically, the enzyme PQQ synthase is involved in biosynthesizing the co-factor PQQ, required for the assembly of the glucose dehydrogenase (GDH) holenzyme, which converts glucose to gluconic acid (Goldstein, 1995). By expression of this gene, synthesis of gluconic acid and thereby phosphate solubilization have been detected in E. coli HB101 (Alaylar et al., 2020). By valuing the involvement of PQQ in secretion of gluconic acid, it is known to be the best mechanism for phosphate solubilization. As reported by Rawat et al. (2020), about 4 - 7 genes (pqqA, pqqB, pqqC, pqqD, pqqE, pqqF, pqqG) depending on the microbes, are involved in PQQ synthesis. All these genes are found to be responsible for dehydrogenase activity and furthermore known to have some vital roles in organic acid production and thereby microbial phosphate solubilization.
To ensure continuous availability of P to crop plants, continual production of organic acids by PSMs is needed as the half-life of organic acids varies from 0.5 - 12 hrs under field conditions. However, if the sorption capacities of soils are high, degradation of organic acid is reported to be significantly low (Oburger and Jones, 2009), thus bioavailability of organic acids and their efficacy depend heavily on sorption processes. Organic acids generally have fewer negative impacts on soil quality parameters such as structure, compaction, and moisture retention compared to inorganic acids produced by microorganisms (Silgado et al., 2020). Furthermore, organic acids by contrast could discharge more H+ than inorganic acids at a given pH which in fact is the determining factor of their efficiency (Li et al., 2016).
Inorganic acids and H2S production
Despite the fact that organic acids are recognized to be the dominant mechanism responsible for phosphate solubilization, the role played by inorganic acids (hydrochloric, nitric, sulfuric, and carbonic acids) is also discussed. However, the effectiveness of inorganic acids and their contribution to phosphate solubilization in soil are comparatively lower. Nitrifying bacteria (Nitrobacter) and sulphur oxidizing bacteria (Acidithiobacillus thiooxidans, Thiomonas intermedia, and Thiomonas perometabolis) are reported to have the ability to produce nitric and sulphuric acids which could convert inorganic phosphates into soluble forms (Khan et al., 2009). The contribution of PSMs towards acidifying the medium is reported when the secrete carbonic acid (H2CO3) during the process of microbial organic matter decomposition. Certain PSMs, during anaerobic decomposition of organic matter, release H2S which could convert insoluble ferric phosphate into soluble ferrous sulphate (Kishore et al., 2015; Rawat et al., 2020).
As reported by Shata et al. (1992), phosphorus content in wheat and maize tissues increased by 3-fold when the plants were treated with a mixture of sulfur and autotrophic sulfur oxidizing bacteria. According to Abd-Elfattah et al. (1992), phosphorus uptake of soybean could be increased by 19.3% when rock phosphate was applied with sulfur, whereas application of rock phosphate with a mixture of sulfur and Thiobacillus thiooxidans could increase the available phosphorus content by nine times than that of untreated rock phosphate. This was further confirmed by El-Tarabily et al. (2006), who also observed the highest growth performance when a combination of sulfur-oxidizing bacteria and sulfur was used. Biofertilizers containing sulfur and Acidithiobacillus were shown to decrease the pH giving a clear indication for Acidithiobacillus mediated production of H2SO4 (Stamford et al. 2003). However, these acids could also act as potential competitors for the binding sites of Al and Fe insoluble oxides which ultimately form chelates. Therefore, the contribution of organic acids for phosphate solubilization is always substantial compared to other contributors.
Proton release from NH4+ (assimilation/respiration)
Proton extrusion is one of the alternative modes of phosphate solubilization mechanisms adopted by PSMs (Parks et al. 1990). Studies with Pseudomonas sp. confirmed that phosphate solubilization could occur without the involvement of organic acids (Illmer and Schinner, 1995). Therefore, release of protons accompanying respiration or NH4+ assimilation could be considered as the most possible cause for phosphate solubilization in the absence of acids (Sharma et al., 2013).
Ammonium (NH4+) ions present in soil are assimilated into amino acids through the sequential actions of several enzymes. Inside the microbial cell, conversion of NH4+ into ammonia (NH3) followed by discharging the excess proton (H+) into the cytoplasm occurs. The proton (H+) released by PSMs lowers the soil pH causing the acidification of the soil solution, which facilitates the phosphate solubilization. However the success of the process depends on the nitrogen sources (Rawat et al., 2020).
The effect of nitrogen source on phosphate solubilization has been investigated by many researches using ammonium (NH4+) or nitrate (NO3-) or both (Walpola et al., 2012). In general, NH4+ as the sole source of N is reported to deliver a greater pH reduction and phosphate solubilization compared to NO3-, owing to the extrusions of protons to compensate for NH4+ uptake (Halder et al., 1991). According to Sharan and Darmwal (2008), higher phosphate solubilization could be achieved when NH4+ is used as a nitrogen source compared to NO3- which is line Scervino et al. (2011) who reported a greater phosphate solubilization by Penicillium purpurogenum and Wang et al. (2020a) who observed the highest phosphate solubilization by multifunctional Bacillus subtilis BPM12 in the presence of (NH4)2SO4 as sole nitrogen source.
Walpola et al. (2012) evaluated the performance of phosphate solubilization of Aspergillus awamori using different media. Results revealed that AYG medium (Halder et al., 1991) which contained the highest amount of nitrogen (1%) provided by (NH4)2SO4 could display the maximum phosphate solubilization. Therefore the presence of NH4+/H+ exchange mechanism which lowers the pH of the surrounding the medium could enhance phosphate solubilization. In fact it has been reported that proton release from NH4+ is the sole phosphate solubilizing mechanism of some microorganisms. Penicillium bilaii and Penicillium fuscum were employed in assessing solubilizing ability of rock phosphate with NH4+ or without N addition, and results revealed that only Penicillium bilaii could solubilize phosphate without external application of N (Asea et al., 1988). Therefore it is certain that phosphate solubilization could be accelerated by proton exchange mechanism when NH4+ is present.
Mechanism of indirect phosphate solubilization
During the nutrient cycling process, microorganisms present in rhizosphere act as a source through their actions in organic matter break down and mineralization, releasing nutrients from cytoplasm to equilibrate with surroundings, and in bursting cell or they could play a role as sink through immobilizing nutrients. By means of dissolving insoluble phosphates, microorganisms could assimilate a substantial portion of P indirectly from the soil solution (Rawat et al., 2020). However, the content of P in PSMs is known to be almost equal to that of in non-PSMs, mainly due to the fact that the P content in microorganisms is correlated with P content in decomposed organic substrates (Kishore et al., 2015). When microbial cells die due to changes in environmental condition, starvation or predation, the P contained in them is released enabling plants and other soil organisms to take up (Kishore et al., 2015). Any unexpected rice in available P content in soil might be due to stress conditions created by the environmental changes like drying and wetting or freezing and thawing which result in microbial cell lysis (Butterly et al., 2009). The P sorption capacity of the soil, in these circumstances, plays a crucial role in making available of P for plant uptake, because a substantial proportion of P is immobilized in the solid phase (Jones and Oburger, 2011). According to Khan et al. (2019), extending the duration of drying and its intensity significantly increases the content of dissolved reactive P in leachate while decreasing the portion of microbial biomass P.
The dynamics of microbial P immobilization are shown to some links with availability of organic matter in the soils and its C : P ratio. If the soil is rich with fresh organic matter inputs, the microbial P content is increased at the beginning, and then decline with their decomposition increasing the P content in soil solution (Jones and Oburger, 2011). However, the quality and the nature of the organic matter, prevailing environment conditions and soil properties decide time elapsed between P immobilization and re-mineralization (Oehl et al., 2001).
Direct oxidation pathway
As proposed by Goldstein (1995), certain bacteria exist in the soil rhizosphere solubilize phosphates through extracellular oxidation of glucose to gluconic acid and further to 2-ketogluconic acid with the secretion of glucose dehydrogenase and gluconate dehydrogenase enzymes respectively. The direct oxidation pathway is much effectively used by gram-negative bacteria to solubilize phosphate compared to that of by gram-positive species. In this connection, gram-negative bacteria are found to be better dischargers of organic acids such as gluconic and 2-ketogluconic acids into the extracellular medium through their sugar metabolism. The organic acids then form chelates of minerals like Ca2+ and Fe2+ and acidify the medium facilitating the dissolution of insoluble phosphates (Krishnaraj and Goldstein, 2001). This direct oxidation pathway mechanism through the production of gluconic acids and glucose dehydrogenase was reported by Song et al. (2008) who observed dissolution of insoluble phosphates by Burkholderia cepacia DA23, Suleman et al. (2018) by Pseudomonas sp. MS16 and Enterobacter sp. MS32 and Linu et al. (2019) by Pseudomonas aeruginosa KR270346. Rhizosphere soils generally contain higher content of gluconic and 2-keto gluconic acids compared to those in the bulk soils, because the rhizosphere soils contain relatively high levels of glucose.
Exopolysaccharide (EPS) production
Apart from organic acids and protons, certain non-organic acid substances which are known as exopolysaccharides (EPSs) could also play some roles in phosphate solubilization. These exopolysaccharides (EPSs) are microbial polysaccharides (homo and heteropolymers of carbohydrates) produced by some bacterial and fungal strains (Ingle and Padole, 2017). They could directly form organo-mineral complexes with metal ions present in the soil in the order of affinity Al3+ > Cu2+ > Zn2+ > Fe3+ > Mg22+ > K+ (Ochoa-Loza et al., 2001). Mendoza-Arroyo et al. (2020) employed Enterobacter sp. ITCB-09, a phosphate solubilizing strain to assess its EPS producing capacity. Findings of their laboratory study revealed that EPS is composed not only carbohydrates, but proteins some hexuronic acids as well. All these compounds might have involved with converting insoluble inorganic phosphates in into soluble forms, through the actions of their ionizable functional groups like carboxyl, carbonyl, or amino. This mechanism could therefore be considered as an example for EPS-mediated phosphate solubilization by some microorganisms.
Yi et al. (2008) employed four bacterial strains (Enterobacter sp. EnHy-401, Arthrobacter sp. ArHy-505, Azotobacter sp. AzHy-510 and Enterobacter sp. EnHy-402) to assess their capacity to produce EPS and thereby phosphate solubilization. According to them, microbial EPS is capable to enhance phosphate solubilization synergistically with the presence of organic acids. However, the success of this synergistic phosphate solubilization (with organic acid and EPS) varies with the source and the relative abundance of EPS in the solution.
Holding free P in the medium, the homeostasis of phosphate solubilization is altered by the actions of EPS which ultimately results in greater P release from insoluble phosphate (Yi et al., 2008). Though a positive correlation between EPS production and P deficiency may exist, it could not be the case in some microorganisms which produce EPSs. However, N supply to the medium could play a greater role in activating EPS production.
Siderophore production
Siderophores (Greek: "Iron carrier") have been identified as low molecular mass (400 - 1,500 Da) chelating compounds. They are secreted by some microorganisms and grass species in response to iron stress in the environment (Rawat et al., 2020). About 500 siderophores produced by different species of microorganisms and plants have so far been identified.
In order to scavenge iron from mineral phases some microbes secrete siderophores. They are then acting in forming soluble Fe3+ complexes which could be taken up by active transport mechanisms (Saharan and Nehra, 2011). Though the role of siderphore in phosphate solubilizing has not been widely studied, it is evident that the most of the PSMs could produce siderophores which obviously could chelate iron from Fe-P complexes in soil (Collavino et al., 2010). Therefore the potential role of siderophores in enhancing phosphate solubilization is obvious, in particular when the dominance of phosphate solubilization over ligand exchange by organic acid is taken into consideration.
Organic phosphate solubilization
The average organic P content in mineral soils varies from 30 to 65% of total P. If the soils contain higher organic matter (> 20 - 30%), the organic P content can be as high as 90% of the total P (Jones and Oburger, 2011). Inositol phosphates, phospholipids and nucleic acids are considered to be the three main organic P compounds available in soil. PSMs solubilize organic phosphates through the secretion of several microbe-derived enzymes (Kumar and Shastri, 2017) such as acid phosphatase (encoded by olpA), alkaline phosphatase (phoD), phytase (appA), phosphonatase (phnX), and C-P lyase (phnJ). There enzymes are found to engage with releasing free orthophosphate from recalcitrant organic P forms.
Phosphatases
Phosphatase enzymes that are secreted by PSMs catalyze the hydrolysis of both phosphoesters and phosphoanhydrides. These phosphatases are either intracellular/membrane bound phosphatases or extracellular phosphatases. The actions of extracellular phosphatases are the key driving force of hydrolysis of organic P (Nannipieri et al., 2011). In this context, relevant experimental evidence elaborating the role of exo- and endo-enzyme is poorly documented.
Phosphomonoesterases (often called phosphatases) are considered to be the most abundant phosphatase enzyme secreted by PSMs. Depending on their pH optima; they could be categorized as acid phosphatases and alkaline phosphatates. Under natural conditions, acid phosphatase/acid phosphomonoesterase is found to be very active in acid soils, while the activities of alkaline phosphatase/alkaline phosphomonoesterase are prominent in neutral to alkaline soils. Furthermore, performance of these enzymes is reported to be greater in rhizosphere compared to that of in bulk soils implying that they could assist in plant P nutrition. However, availability of phosphatase enzyme is determined by several factors such as mineral composition, organic matter content and pH of soil.
Not only PSMs, but plant roots could also secret acid and alkaline phosphatases though the production quantities of alkaline phosphatases are low. Though it is hard to distinguish phosphatases based on their origin, it has been reported that both acid and alkaline phosphatases secreted by PSMs provide with greater affinities for organic P compounds than those derived from plant roots (Sharma et al., 2013). Furthermore, alkaline phosphatase performs better than acid phosphatase in terms of enzyme activity. Jarosch et al. (2015) reported that 90% of total organic phosphorus was hydrolyzed by alkaline phosphatases enabling plants to uptake phosphorus. Phosphatase activity is reported in many PSMs like Serratia sp. and Pseudomonas sp. (Blanco-Vargas et al., 2020), Trichoderma sp. (Bononi et al., 2020), Pseudomonas sp. and Pantoa sp. (Chen and Liu, 2019).
As depicted in Fig. 2, organic P in the extracellular environment is hydrolyzed by the action of phosphatase enzymes, facilitating plants to uptake inorganic P (Nannipieri et al., 2011). Its activities have been shown to be induced under P deficiency conditions.
Alcaligenes faecalis, a phosphate solubilizing bacteria is reported to deliver the maximum alkaline phosphatase activity (93.7 U·mL-1) under optimized conditions. As reported by Behera et al. (2017a), partially purified alkaline phosphatase exhibited the maximum activity of 96.53, 97.99, and 96.51 U·mL-1 respectively at pH 9.0, temperature of 45℃ and substrate concentration of 1.75 mg·mL-1. Similarly phosphate solubilizing bacteria Serratia sp. is reported to produce acid phosphatase with the maximum activity of 85.6, 97.87, and 92.7 U·mL-1 respectively at pH 5.0, temperature of 45℃ and substrate concentration of 2.5 mg·mL-1 (Behera et al., 2017b). The concentration of phosphatase enzyme secreted by PSMs varies with the type of carbon and nitrogen source of the medium. Behera et al. (2017a) investigated the impact of carbon and nitrogen supplementation on phosphatase enzyme production and reported that some carbon and nitrogen sources could not enhance the production of phosphatase. Bacterial isolates Alcaligenes faecalis and Serratia sp, are shown to prefer glucose and ammonium sulphate respectively as the carbon and nitrogen source to produce the maximum amount of alkaline and acid phosphatase (Behera et al., 2017a, b).
Phytases
Breaking down of organic P from phytate to inorganic P enabling plant uptake is performed by a group of enzymes called phytases (myo-inositol hexakisphosphate phosphohydrolases). Phytic acid/phytate/inositol hexakisphosphate, a saturated cyclic acid is identified as the key form of P stored in many soils and plant tissues. Though phytate is not available for direct plant uptake, it is found to be the major form of organic P which accounts for approximately 60 - 80% of organic P in soil. Furthermore, it presents in cereals, pollens, oilseeds, edible legumes and nuts at 1 to 5% (by weight) (Singh and Satyanarayana, 2011).
Though plants, microorganisms and some animal tissues are known to be the main sources of phytases, regulation of phytate mineralization in soil is heavy dependent upon the availability of microorganisms. Investigations conducted with some bacteria, yeast and fungi have proved that they are capable of converting organic P into inorganic P making P available for plant uptake through the production of extracellular phytase. Fig. 3 depicts the mechanism through which PSMs in the rhizosphere hydrolyze phytate and make P available for plant uptake (Richardson and Simpson, 2011).
A wide range of variations in phytase activity was reported by Ramesh et al. (2011) who inoculated several Bacillus isolates to the soil despite the increments in available P content is soil solution. Therefore it is obvious that the phytase activity has positive impacts on P mobilization. According to them, the seed yield of soybean plants has increased with the inoculation of different Bacillus isolates which facilitated the availability of higher P content through their enzyme activities. A significant positive correlation between phytase-driven available P content in rhizosphere and P content in soybean seeds has been observed giving a clear indication that inoculation of Bacillus isolates and phytase enzyme has an impact on P mobilization and thereby plant uptake of P.
Phosphonatases/C-P lyases
Phosphonates are recognized as ubiquitous organophosphorus substances having a characteristic carbon-phosphorous (C-P) bond which is known to be chemically inert and hydrolytically stable. Some PSMs are capable of metabolizing these phosphonate substances through the production of phosphonatases/C-P lyases improving the P availability to plants. Therefore, phosphonate hydrolases are recognized as ‘phosphonatases’ (Kamat and Raushel, 2013).
The substrates which are good for the production of phosphonatases generally have an electron withdrawing b-carbonyl group that enables bond delocalization and heterolytic cleavage of the C-P bond. Phosphonopyruvate (PnPy), phosphonoacetate (PAA) and phosphonoacetaldehyde (Pald) are some of the enzymes that could hydrolyze phosphonate substrates (Fig. 4).
Organic phosphate solubilization through C-P lyases is found to be minor; due to low enzyme activity in soils where relevant substrates are lacking (Selvapandiyan and Bhatnagar, 1994). However, some bacteria such as Burkholderia, Bacillus, Pseudomonas, Enterobacter, Acinetobacter and Rhizobium (Teng et al., 2019) and some endophytic fungi including Aspergillus, Penicillium, Piriformospora, and Curvularia (Mehta et al., 2019) are known display the C-P lyases activity.
Conclusion
Available P is known to be a limiting factor more regularly in tropical soils where it is usually present as insoluble metal chelates. Furthermore, a considerable portion of applied phosphate fertilizers to soils is fixed as insoluble phosphate sources. Consequently, application of P fertilizers is become a regular practice, though it is costly and environmentally undesirable. At a stage where world agriculture faces numerous challengers to produce food for the increasing population, dependency on synthetic fertilizers to enhance the yield is hard to avoid. However, repeated use of chemical fertilizers often causes negative impacts on the environment. In this context, employment of organic tactics such as use of biofertilizer is underway as such methods are believed to sustain the soil fertility and plant productivity while protecting the natural and agricultural ecosystems. PSMs convert both unavailable organic and inorganic P into available P through mineralization and solubilization to satisfy the requirements of plants. The key mechanism involved in P solubilization is the production of organic acids having low molecular weight and discharge them into the adjacent soil environment. Apart from this, production of inorganic acids and H2S, exopolysaccharides, siderophores, protons, and hydroxyl ions are also attributed to inorganic phosphate solubilization. Organic P compounds undergo mineralization which is facilitated by enzymes especially acid and alkaline phosphatases, phytases and phosphonatases/C-P lyases. Though novel PSMs with the potential use as bio-inoculants to promote soil fertility have been discovered, additional researches are still needed to evaluate their performance under diverse ecological niches. Furthermore continuous exploring of new PSMs which manifest numerous growth promoting features of agricultural plants is also needed.