Introduction
Vegetables are horticultural crops that are grown annually or perennially, and are essential for human nutrition due to their high dietary fiber content and bioactive nutrients, which can be consumed either raw or cooked (Olatunji and Afolayan, 2018). These nutrients and non-nutrient molecules lower the risk of chronic health conditions like cardiovascular disease, diabetes, and obesity (Chen et al., 2018). Around 10,000 plant species are classified as vegetables worldwide based on common features (Barthlott, 2005). Ülger et al. (2018) categorized vegetables into green vegetables (e.g., spinach, lettuce, curly lettuce, celery, and broccoli) and root vegetables (e.g., carrot, beet, turnip, fennel, onion, and radish). In 2020, global vegetable production was 1,248 million tons, with China as the top vegetable-growing country, followed by India, with 30% and 14% of global production, respectively (Food, 2021). In 2019, the highest-production vegetable crops in Korea were Chinese cabbage, onion, radish, potato, green onion, tomato, sweet potato, cabbage, cucumber, pumpkin, garlic, and peppers, which are consumed as side dishes in more than 83% of Korean diets (Nieuwsbericht, 2021). Upland field farming mechanization rates in Korea 99.8, 5.0, 67.6, 95.8, and 13.3% for plowing and harrowing, sowing and transplanting, vinyl covering, pest and disease control, and harvesting, respectively (Yu et al., 2015). In addition, the overall percentage of mechanized upland farming in Korea is increasing, with were 45.5, 55.7, 56.3, 58.3, 60.2, and 61.9% for the years 2009, 2012 - 2013, 2014 - 2015, 2016, 2017, 2018 - 2019, and 2020 - 2021, respectively (MAFRA, 2023).
Transplanting vegetables entails transferring seedlings from their original growing medium (nursery pots) to a new growing medium (the main field) to enable them to grow to the desired size for yield production (Diao et al., 2016). For proper transplanting, several transplanters and tools are used, and their designs depend on the type of seedling crop (Kumar and Rahman, 2011). Seedling production aims at producing a plant that has a well-developed top and root in a single location, such as Styrofoam or plastic trays filled with appropriate medium for the growth of up to four to five genuine leaves (Russo, 2012). In the field, transplanters hold seedlings in trays or pots, make a furrow in the soil to a desired depth, release seedlings from a seedling tray, adjust the distance between seedlings, place the seedlings vertically in the furrow without damaging them, cover the seedlings with soil, and firm the soil (Dihingia et al., 2016). In the vegetable production value chain, seedling transplanting is a crucial operation that is laborious, time-consuming, and ineffective when performed manually (Khadatkar et al., 2018). Furthermore, during the agricultural peak season, labor shortages cause delays in transplanting, resulting in a drastic reduction in yields (Bhatt et al., 2016). Therefore, mechanized vegetable production, such as the use of vegetable transplanters, might be a solution to address these vegetable cultivation challenges (Diao et al., 2016).
Transplanter performance evaluation during the planting process is critical, as it serves as an indicator of effective field efficiency and transplanting efficiency analysis (Lee et al., 2018). The objective of this research was to review the currently available vegetable transplanters and the previous kinematic analyses of their major transplanting mechanisms. This study will also provide useful information on the capabilities, efficiency, and appropriate mechanisms of vegetable transplanters, which will guide future researchers and manufacturers to improve vegetable-transplanter designs. The classification of vegetable transplanters is first described, followed by detailed explanations of the transplanting mechanisms involved in vegetable-transplanting processes.
Classification of vegetable transplanters by level of mechanization
The vegetable transplanters used in recent decades can be classified as handheld, semi-automated, and fully automated transplanters based on their operating principles (Sharma and Khar, 2022). The main structural components of vegetable transplanters are seedling nursery trays or tray holders, a picking unit, a conveying unit, and a planting unit for the planted seedling (Kumar and Rahman, 2008). Fig. 1 shows the general classification of vegetable transplanters.
Handheld transplanters are operated by human hand power (Khadatkar et al., 2018), while semi-automatic and fully automatic transplanters are propelled by an engine and require an operator to walk or sit alongside them (Pandey, 2006). Among automatic transplanters, walking types are operated by steering handles, while tractor-drawn types must be attached to a tractor and require an operator; all of them require workers to manually feed seedlings into the planting device (Rahul et al., 2019). The fully automated vegetable transplanter is designed to provide continuous seedling feeding while transplanting automatically, which reduces the transplanting duration and human labor (Han et al., 2021). In addition to field conditions, crop type, and other operating parameters, the transplanting system is evaluated based on plant-to-plant spacing, planting depth, transplanting efficiency, furrow closure, angle of inclination, and misplanting (Khadatkar et al., 2021). The effective field efficiency and transplanting efficiency of different transplanters have previously been calculated. Table 1 shows the effective field efficiency and transplanting efficiency for different types of vegetable transplanters.
Handheld transplanters
Handheld transplanters are powered by human hands and have a handle, hopper, seedling delivery tube or hollow pipe, jaw opening lever, and jaw mouth as major components. (Khadatkar et al., 2018). For transplanting, the jawed mouth penetrates into the soil by applying a little force on the clutch while holding a handle with the upside movement of the clutch lever, which conveys the seedling into the furrow and covers it with the surrounding soil to a vertical planting state of 2-cm depth, following the pushing, punching, and earthing mechanisms (Sharma and Khar, 2022). A handheld transplanter was tested on several vegetable crops; the findings revealed that it operated properly, with no misplantings, and a negligible rate of tilted planting, on 82.30% field efficiency (Kaushik et al., 2020). Fig. 2 illustrates the component parts of the handheld transplanter, as well as the sequence of transplanting steps. Table 2 describes the performance comparison of handheld and manual transplanting.
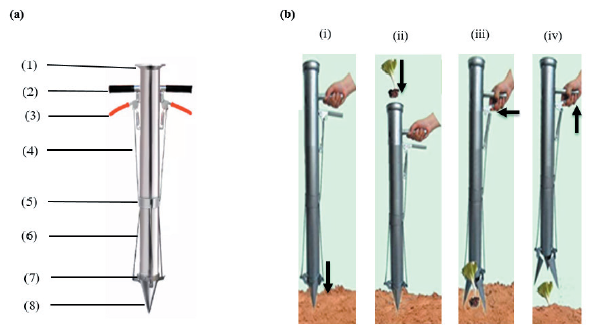
Fig. 2. Hand-held vegetable seedlings transplanter parts (a): seedling hopper (1), Rubber handle (2), pull handle (3), pull wire (4), sliding tube (5), stainless steel body (6), depth lever (7), triangle cup (8), and seedling transplanting steps (b): insert the transplanter into the soil by pushing (i), put the seedling into a transplanter cylinder tube by earthing (ii), hold the handle by punching (iii), and lift the transplanter (iv).
Table 2. Performance comparison between handheld and manual transplanting methods (Rasool et al., 2020).![]() |
Semi-automatic transplanters
Semi-automatic vegetable transplanters consist of an engine that generates power, a transmission system that distributes the power to the driving wheel and transplanting device, a seedling cylinder where seedlings are manually placed, a transplanting device that plants the seedlings supplied from the seedling cylinder into the soil, a control section that manages the operation of the transplanter, and a plant-spacing control device (Sri et al., 2022). The operator manually feeds seedlings into the hopper, after which point the transplanting is automated. The tractor-drawn, semi-automatic vegetable transplanters contain a seat for the worker, who loads the seedlings into the planting unit (Khadatkar and Mathur, 2022). Fig. 3 shows the structure of a semi-automatic seedling transplanter.
The seedlings are kept in a seedling holder before being fed into the planting unit. The seedlings are metered at the specified seeding rate, then transferred to the furrow at predetermined intervals and covered with the surrounding soil (Kumar and Rahman, 2008). A performance evaluation comparing a semi-automatic vegetable transplanter with manual transplanting showed that the effective field efficiency of semi-automatic transplanters was 0.026 ha·h-1, with 81% transplanting efficiency proportional to the speed of the transplanter (Sharma and Khar, 2022). Tables 3 and 4 show the comparison between semi-automatic pepper transplanting and manual transplanting, and the field performance of a semi-automatic transplanter, respectively.
Fully automated transplanters
A seedling-feeding device, combing device, inserting ejecting-type seedling extraction device, plate-turning device, seedling-conveyor device, seedling-separation device, planting device, empty-tray recovery device, and suppression device are the main components of a fully automated transplanter, as shown in Fig. 4. The fully automatic transplanter moves through the transmission system, driving the seedling-separation device, planting mechanism, air compressor, and generator (Wen et al., 2021).
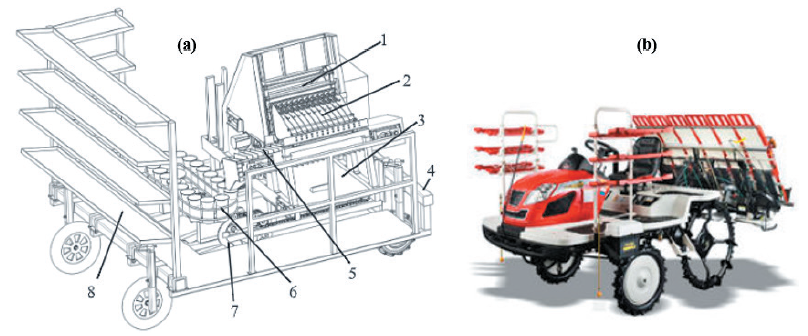
Fig.4. Overall structure of a fully automatic transplanter: major components (a): seedling feeding device (1), combing device (2), empty tray recovery device (3), chassis (4), seedling conveyer device (5), seedling separation device (6), planting device (7), Plug seedlings rack (8), and fully automatic transplanter (b).
Table 4. Field performance of a 2-row semi-automatic transplanter developed by Punjab Agricultural
University (PAU) in 2004 (Abdulai, 2020).![]() |
The seedling-feeding device carries plug seedlings to a stationary position in order to supply them to the seedling-extraction device. The seedling-extraction cylinder steers the mandrels through the bottom holes of the tray and the seedling holes, allowing an entire row of plug seedlings to move into the corresponding grids of the conveyor. Whenever the thimble is retracted, the plate-turning device inhibits the seedlings from being handed back. Plug seedlings are dropped one by one into the separation part via the seedling-dropping siphon as they are moved forward on the conveyor belt in an orderly and intermittent manner. The separation parts spin to carry the plug seedlings from the left and right seedling pots, and plant them. Therefore, the suppression wheel impedes the soil on both sides of the plug seedlings. The empty tray recovery conveyer and empty tray palletizing part gather and load the empty trays during the transplanting process. Fig. 5 and Table 5 show the working-principle steps and performance of automatic transplanters, respectively.
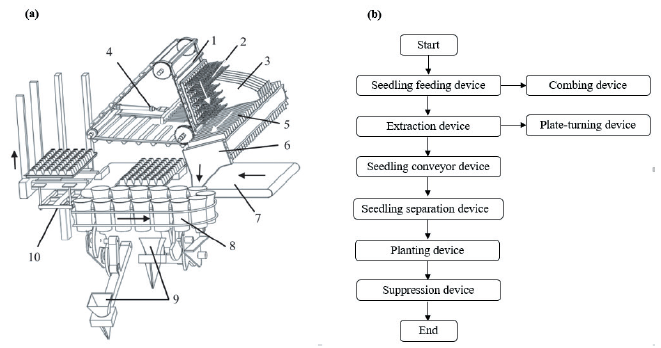
Fig. 5. Schematic diagram of a fully automatic transplanter (a): seedlings feeding device (1), plug seedlings (2), combing device (3), inserting ejecting-type seedling extraction device (4), seedling conveyor belt (5), seedling-dropping funnel (6), empty tray recovery belt (7), seedling separation device (8), planting device (9), empty tray palletizing device (10), and working principle of an automatic transplanter (b) (Wen et al., 2021).
Table 5. Performance evaluation of automatic and manual chili seedlings transplanter (Han et al., 2019).![]() |
Vegetable transplanting mechanisms
The main functional components of vegetable-transplanting mechanisms are seedling nursery trays, and picking, supply-system and dibbling devices that are all synchronized together (Islam et al., 2020). The overall vegetable transplanter structure consists of a picking mechanism that picks multiple vegetable seedlings from a nursery tray simultaneously and places them on the conveyor of the supply mechanism for continuous seedling supply to the dibbling mechanism, which plants them into the soil, covers them with the surrounding soil, and firms the soil up. Fig. 6 describes the overall structure of an automatic vegetable transplanter and its mechanisms.
Picking and dibbling are the major vegetable-transplanting mechanisms, and are mainly categorized into bar-based linkages and gear-driven mechanisms, each of which can be subdivided into several unique mechanisms. The bar-based linkage picking type is further classified into pin and pushing seedling-extraction types, whereas the gear-driven picking type is further classified into planetary and spur gear train types. Furthermore, the bar-based linkage dibbling type is divided into the wheel and cam linkage types, whereas gear-driven dibbling types are divided into planetary and spur gear train types, as described in Fig. 7.
Picking mechanism
The automatic transplanter picking mechanism is an essential component designed to extract seedlings from a nursery tray and discharge them onto the planting device. The reliability and effectiveness of the transplanting process are determined by the synchronization of the seedling auto-picking mechanism with the dibbling mechanism, which enables the timely planting of the desired quantity of seedlings. The common components of the picking type are a picking stand to hold and maintain the positions of all elements in the supply unit, manipulators to move the grippers to the conveying device, grippers to release the seedlings, and a gear box to help the cam and crank rotation. The working space of picking-mechanism work depends on the height of the picking stand and the distance from the seedling tray (Han et al., 2019).
Since the 2000s, researchers have been motivated to develop several versions of automatic picking mechanisms based on the operation method and mechanical linkages of picking mechanisms such as gears and cams (Yu et al., 2019). Choi et al. (2002) invented the seedling picking mechanism for vegetables using a five-bar mechanism made up of a fixed slot, driving link, driven link, connecting links, and a slider, with a success rate of 80.0 - 94.4%. In 2005, the pin-type seedling pick-up mechanism was developed. The device featured a set of pins that could raise the seedling from the tray once and then drop-release it into a defined position such as a furrow, belt conveyor, or tray (Kumar and Rahman, 2008). Table 6 describes the comparison between the existing picking mechanisms for vegetable transplanters.
Transplanting (dibbling) mechanism
The seedlings are collected from the conveyor and dug into the soil at the desired depth in an upright orientation by the transplanting or dibbling mechanisms. The dibbling mechanism comprises three major components: a primary arm, a connecting arm, and dibbling hoppers. Primary arm rotation allows the dibbling hoppers to move up and down in an oval-shaped trajectory. The connecting arm aids in the connection of hoppers, and the hopper collects seedlings and places them inside the soil along their working trajectory. Better crop growth and yield depend on a proper dibbling mechanism (Dihingia et al., 2016). Furthermore, the velocity of the hopper and the forward speed of the transplanter are essential for successful seedling transplanting. In addition, the vibration, power usage, and lifespan of the transplanter affect the acceleration of the mechanism (Iqbal et al., 2021).
In 1997, a static cassette-type mechanism was developed (Brewer, 1997), followed by the development of the transplanting disc or split cone cup-type dibbling device several years later (Herrison et al., 1988). Researchers from China and Korea started to develop the dibbling mechanism in the 20th century by including a rotating mechanism for the high-speed transplanting of seedlings in an effort to fabricate a small-scale, inexpensive transplanter. A rotating mechanism with a bar link and cam was developed by Park et al. (2005) for this train of invention, and later spur gear trains with the dibbling hopper-type mechanism were added for a planting rate of 45 seedlings per minute. Table 7 summarizes the existing transplanting mechanisms for vegetable transplanters.
Design variables of major mechanisms
The design of the transplanter components and proper operation handling are critical to effective and efficient seedling transplanting (Khadatkar et al., 2020). The most important considerations are the appropriate design structures of variable and fixed-parameter combinations for both picking and dibbling mechanisms. To meet the seedling picking trajectory requirements, the dimension combination of a manipulator, grippers, and a picking stand should be considered for the picking mechanism (Islam et al., 2020). In addition, a proper dibbling mechanism is determined by arm link lengths, gear diameters, and gear tooth numbers (Iqbal et al., 2021). Table 8 summarizes the design variables for existing picking and dibbling mechanisms for vegetable transplanters.
Kinematic analysis of vegetable-transplanter mechanisms
Kinematic analysis is the preliminary design stage that aids to determine the kinematic parameters of mechanical components in order to enhance a specific mechanism (Raza et al., 2018). This type of analysis entails developing and explaining equations for the path motion, velocity, and acceleration of machinery parts, as well as the relation between their inputs and outputs, in order to comprehend the mechanical operations that can be used to validate the design parameters (Reza et al., 2021). Furthermore, kinematic analysis provides valuable insights into the design and performance of transplanters, and can assist in identifying areas for improvement in terms of efficiency, accuracy, comfort, and durability (Pérez-Ruiz and Slaughter, 2021). Analytical techniques, CAD systems, and computer methods such as the vector loop model are applied to establish the workspace trajectory of the moveable components of transplanters in accordance with the agronomy crop requirements (Hwang et al., 2020).
There have been several studies conducted on vegetable transplanters to improve their design, performance, and efficiency. Iqbal et al. (2021) proposed a kinematic study of a hopper-type dibbling mechanism on a 2.6 kW, two-row pepper transplanter in order to optimize its design and performance. The study focused on the motion and behavior of the mechanics and components of the transplanter, including the dibbling hopper and planting units. The path, velocity, and acceleration of each component were determined by simulating and analyzing the motion of transplanter components. According to the findings, the hopper-type dibbling mechanism provided consistent and precise seedling positioning, and the hopper effectively fed the dibbler and planting unit with seedlings. Islam et al. (2020) performed a kinematic analysis, virtual modeling, and validation testing of a clamp-type picking mechanism on an automated pepper transplanter. The proposed picking mechanism mainly consisted of a manipulator with five grippers and a picking stand. A virtual model simulation and high-speed photographic experiments were carried out to determine the kinematic properties of the picking device. The results suggested that picking device size had an effect on the position, velocity, and acceleration of the gripper, and that low velocity and acceleration may aid in the success of pepper planting by protecting seedling damage. Zhang et al. (2011) conducted a kinematic study of a cantilever-cup vegetable transplanter to determine the seedling velocity equation during operation. The ground wheel, planting wheel, and planting apparatus were designed based on theoretical opinions, and the performance indices of the prototype were evaluated through trials conducted in a controlled laboratory setting. Seedling damage was 2.19%, indicating that the machine’s productivity was at or above the required level. Reza et al. (2021) proposed modeling a gear-driven rotational planting mechanism with a vector loop for a six-row self-propelled onion transplanter. A kinematic analysis was used to improve the path trajectory through computer simulations, and field tests were conducted to determine the link combinations and evaluate the planting mechanism. The maximum power for the planting mechanism was determined to be 35.4 W during the field test, with transplanting rates of 60 and 120 seedlings per minute for the single- and double-planting mechanisms, respectively. Hu et al. (2014) designed a high-speed seedling transplanting robot with a 2-degrees-of-freedom (DOF) parallel translation mechanism and a pneumatic manipulator using the inverse kinematics of the parallel mechanism. The transplanting robot was simulated using rigid-body kinematics and dynamics, and the resulting data included power and torque variation curves, as well as displacement, velocity, acceleration, and jerk variation curves for the manipulator and two active arms. The findings demonstrated the capability of kinematic analysis to validate both the structural design, dimension synthesis, and trajectory planning required for successful seedling transplantation. Fig. 8 shows the high-speed plug-seedling transplanting robot with a 2-DOF translational parallel mechanism. Sun et al. (2016) developed a wide narrow-pot seedling transplanting mechanism (WPST) with noncircular bevel gears using a kinematic model based on displacement and orientation data known as D–H transformation matrices. The simulation model was used to validate the outer pitch curves of noncircular bevel gears. The study’s findings revealed that the radius vectors of the outer pitch curves, the length of the seedling paw, and the planting arm installation angle influence all had an effect on the contour of the transplanting trajectory. Fig. 9 shows the structure of the potted seedling transplanting mechanism.
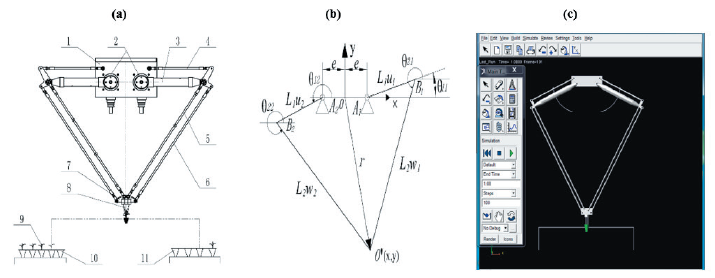
Fig. 8. Sketch of plug seedling transplanting components (a): frame (1), servomotor (2), inner active arm (3), outer active arm (4), inner followed arm (5), outer followed arm (6), moving platform (7), manipulator (8), plug seedling (9), supplying tray (10), planting tray (11); kinematic analysis model of 2-degree of freedom (DOF) transplanting mechanism (b) and trajectory simulation of transplanting (c) (Hu et al., 2014).
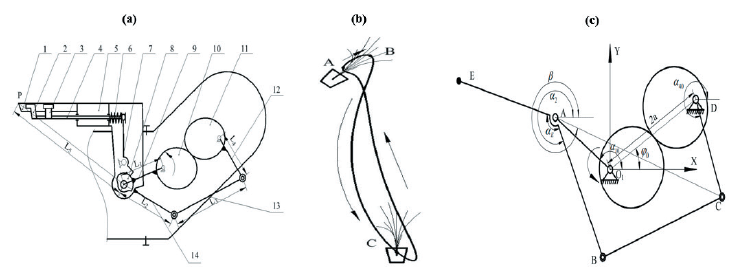
Fig. 9. Structure of the potted seedling transplanting mechanism (a): seedling clip (1), U-shaped block (2), seedling-pushing roll (3), seedling-pushing rod (4), transplanting arm (5), seedling-pushing spring (6), fork (7), cam (8), driving crank (9), driving elliptical gear (10), driven elliptical gear (11), driven crank (12), pendulum rod (13), connecting rod (14); working trajectory of seedling picking mechanism (b) and kinematic analysis of double crank mechanism (c) (Sun et al., 2016).
Table 9 includes examples of kinematic analysis for various picking and dibbling mechanisms published in the literature, as well as their related field applications. Accordingly, previous studies have highlighted that using sensors and control systems can significantly improve the accuracy of vegetable-transplanting mechanisms. This can lead to reduced misplanting; maintenance of plant spacing, planting depth, and specific planting depth; protection from seedling damage, increased productivity, and higher overall crop yields.
Trends in research have also focused on improving the speed and efficiency of vegetable transplanters so that they can cover more field area in less time by optimizing the mechanical design, reducing the weight, improving the power source, and enabling adaptability to the different crop types and soil conditions. Furthermore, research has looked at ways to lower the cost of vegetable transplanters, such as by using lighter and less expensive materials, optimizing the design for mass production, and investigating alternative power sources, such as human-powered or electric transplanters. These studies emphasize the importance of ongoing research and development in the field of vegetable transplanters, which play an important role in the success and sustainability of vegetable farming operations.
Conclusions
This study reviewed the currently available vegetable transplanters and previous kinematic analyses of their corresponding mechanisms. The operating principles, effective field efficiency, and transplanting efficiency of each type of vegetable transplanter were described based on the literature. Fully automatic vegetable transplanters were the most effective, with an effective field efficiency of 0.093 ha·h-1 and a transplanting efficiency of 92.6%. On the other hand, semi-automatic transplanters had an effective field efficiency of 0.026 ha·h-1 and a transplanting efficiency of 81%. For the handheld transplanters, the effective field efficiency was 0.0243 ha·h-1, and transplanting efficiency was 56.4%. This indicates that the development of automatic transplanter designs has improved the efficiency of vegetable transplanting significantly. Furthermore, under different field conditions, the transplanting performance of handheld, semi-automatic, and fully automatic transplanters—represented by the proportion of misplanting—was found to be 0, 3 - 8%, and 4.9%, respectively.
The main vegetable-transplanting mechanisms were picking and dibbling, which were further divided into bar-based linkages and gear-driven mechanisms. Each mechanism was then divided into a number of unique mechanisms. A successful vegetable-transplanting operation requires careful consideration of the picking mechanism trajectory, the hopper velocity, and the transplanter forward speed. The appropriate operational component dimensions, the combination of link bars and gears, and the degree of freedom were previously determined by several researchers using a variety of methods including analytical modeling, software simulation, and kinematic analysis to ensure transplanting success.
According to recent studies, the most suitable recommended combinations of gripping techniques for clamp and sliding are 4- and 5-bar-based linkages with 1 and 2 degrees of freedom. Furthermore, rotating-type, gear-driven dibbling mechanisms were recommended for their capability to provide an adequate planting speed of 45 seedlings per minute. Future research should concentrate on minimizing the distance between the seedling supplies and picking device in order to maintain the seedling flat pick-up trajectory, and controlling the free-fall of seedlings from the discharging mechanism without damaging them.
Acknowledgments
This work was carried out with the support of “Cooperative Research Program for Agriculture Science and Technology Development (PJ01606101, Combined work type high-performance field crop precision planting & transplanting technology development)” Rural Development Administration, Republic of Korea.
Authors Information
Eliezel Habineza, https://orcid.org/0000-0003-0364-420X
Mohammod Ali, https://orcid.org/0000-0002-1822-3005
Md Nasim Reza, https://orcid.org/0000-0002-7793-400X
Jea-Keun Woo, https://orcid.org/0000-0001-8045-8908
Sun-Ok Chung, https://orcid.org/0000-0001-7629-7224
Yaxiu Hou, https://orcid.org/0000-0002-7449-4547