Introduction
Maize (Zea mays L.) holds significant importance as a staple food source and serves as a valuable model organism for genetics and biological research (Buckler et al., 2006). Its diverse applications in the food, feed, and industrial sectors underscore its significance in agriculture on a global scale (Ranum et al., 2014). Moreover, the value of maize is further enhanced by its rich nutritional content in the aleurone layer of the endosperm, such as essential amino acids, vitamins, and minerals (Kumar et al., 2023). The nutritional composition in maize makes it a highly potential crop, contributing to improve food security and addressing malnutrition challenges (Shiferaw et al., 2011).
Maize undergoes a complex developmental process in the endosperm after fertilization of the central cell (Becraft, 2001; Leroux et al., 2014). This process involves the differentiation of various distinct cell types including starchy endosperm, aleurone layer, and so on. The starchy endosperm acts as the primary storage tissue, accumulating large quantities of starch (Lopes and Larkins, 1993; Obadi et al., 2023). On the other hand, the aleurone layer plays a critical role in seed germination and development, serving as a reservoir for minerals and high quality proteins (Jerkovic et al., 2010; Becraft and Yi, 2011). While most maize varieties typically possess a single aleurone layer, there have been rare occurrences where maize exhibits multiple aleurone layers. One of such instance was observed in a landrace Coroico, which has been documented to contain two to six aleurone layers (Wolf et al., 1972). This unique characteristic contributes to elevated protein content, as the multiple layers have an additive effect on protein accumulation. Furthermore, the presence of multiple aleurone layers in maize resources was associated with a higher nutritional value compared to those with a single aleurone layer (Welch et al., 1993; Paulsmeyer and Juvik, 2023).
The cereals possessing aleurone layers, such as rice and wheat, variations in layer thickness based on location have been observed (Xiong et al., 2013; Wu et al., 2016). However, due to the comparatively larger size of maize seeds in relation to other cereal crops, in most cases partial observations of the aleurone layer have been conducted in maize. Therefore, diverse studies are required to uncover the patterns of aleurone layers across the entire cross-section in various maize genetic resources.
Microscopic analysis of plant tissues plays an important role in unraveling their intricate structures and compositions, thereby enhancing our understanding of a multitude of biological processes. This analysis can be achieved through methods such as partial observation via hand sectioning and comprehensive cross-section utilizing a microtome. While hand sectioning expeditiously facilitates the examination of specific regions, its proficient execution necessitates a certain level of expertise. Conversely, microtome-based sectioning yields high-resolution images, excelling in visualizing softer tissues (Dey, 2023). However, this preparatory process involves embedding the sample in an appropriate medium, meticulous alignment, and precise sectioning to obtain the desired outcomes. The intricacies of this procedure can be time-intensive and require specialized skills, often restricting its application to well-equipped laboratories or skilled researchers. With advancing technology, there may be efforts to develop more accessible and user-friendly methods to obtain detailed cross-sectional information, catering to a broader range of researchers and facilitating extensive research.
In this study, we present a modified method for efficient microtome cross-sectioning that enables the acquisition of entire cross-sections of maize kernels. This technique not only saves time and effort but also facilitates comprehensive investigations of critical properties within the aleurone layer. The significance of this work lies in its potential to accelerate research on maize development, nutritional qualities, and overall grain functionality, contributing to the advancement of maize research and its agricultural applications.
Materials and Methods
Plant materials
Six maize accessions were used in this study including B73, Mo17, the thk1 mutant, two South American landraces, and a Korean landrace (Table 1). Plant materials were selected for their typical aleurone phenotypes based on previous studies. B73 and Mo17 are widely used wild-type accessions having a single aleurone layer and a reference genome (Schnable et al., 2009). The multi-aleurone phenotype mutant, thk1 reference allele was included for observing the multi-aleurone layer (Yi et al., 2011). The landraces were selected for their multi-aleurone phenotype either reported in a previous study (Wolf et al., 1972) or in our previous observation. The thk1-R seeds were acquired from the MaizeGDB (maize genetics and genomics database, https://www.maizegdb.org/). Two South American landraces, Coroico and Peru 442, were obtained from CIMMYT (Centro Internacional de Mejoramiento de Maíz y Trigo, International Maize and Wheat Improvement Center, https://www. cimmyt.org/). The seeds for B73, Mo17, and a Korean landrace were obtained from the National Agrobiodiversity Center of Korea (http://genebank.rda.go.kr).
Microscopic analysis
The maize samples were longitudinally halved using a razor blade and were fixed using formaldehyde for a duration of 4 hours. After fixation, the samples underwent a series of dehydration steps utilizing ethanol, concentrations of 70, 80, 90, and 100%, 30 minutes for each. The samples were then subjected to an hour treatment with xylene. Xylene functioned as a clearing agent, effectively displacing ethanol and enhancing sample transparency for the following processing. Subsequently, the dehydrated and cleared samples were embedded in paraffin, which were melted at 60℃ in a dry oven. These samples were adhered to on wooden molds with additional liquid paraffin. Utilizing a microtome (HistoCore Biocut, Leica Biosystems, Germany), the paraffin-embedded specimens were sliced into 30 μm thickness. These delicate sections were then carefully placed onto glass slides. Upon completion of these preparatory steps, the mounted sections on the glass slides were subjected to staining using toluidine blue. Subsequently, the stained sections were subjected to the microscope (Olympus BX51, Olympus, Japan) and camera (Progress Gryphax, Jenopitk, Germany) to investigate the property of the aleurone layer (Fig. 1).
Results and Discussion
A rapid and simple sectioning technique for visualizing the aleurone layers
Using the presented method, we achieved a comprehensive visualization of the cross-section in maize. In this protocol, we were able to achieve substantial time savings compared to the previous micro-technique by Sylvester and Ruzin (1994). In the conventional method, hard seeds typically necessitated over 24 hours of fixation, followed by subsequent dehydration in ethanol, xylene, and paraffin, which added an additional 10 hours to the process. By integrating these steps, we successfully reduced the total processing time to a maximum of 8 hours. The aleurone layer in the B73 inbred line consisted of a single layer (Fig. 2). However, noteworthy irregularities were observed in the central region close to the silk scar (Fig. 2C), where indentations were made because of less filled floury starch endosperm. Conversely, Mo17 inbred line exhibited a consistent and uniform single layer pattern of aleurone (Fig. 2G and H). The results indicating irregular patterns in aleurone among maize genetic resources with a single layer emphasize the need for comprehensive investigations into other resources possessing multiple aleurone layers. To obtain an entire cross-section, we dissected in 30 μm slices with microtome which was too thick to get a high resolution and high magnitude image, however with a low magnitude less than 10×, we successfully observed the whole cross section of a kernel.
Fig. 2
Seeds and aleurone phenotypes in inbred lines with a single aleurone layer. (A) Kernel appearance of B73, (B) longitudinal cross-section of a B73 kernel, (C, D) the aleurone layer of B73 sections stained with toluidine blue and observed by microscope. (E) The kernel appearance of Mo17, (F) longitudinal cross-section of a Mo17 kernel, (G, H) the aleurone layer of Mo17 sections stained with toluidine blue, observed under a microscope. Red arrows indicate points of microscopic observation, the white scale bars represent 0.5 cm, and the black scale bars represent 50 μm. The yellow arrow in (C) indicates the sporadic double aleurone layer of B73.
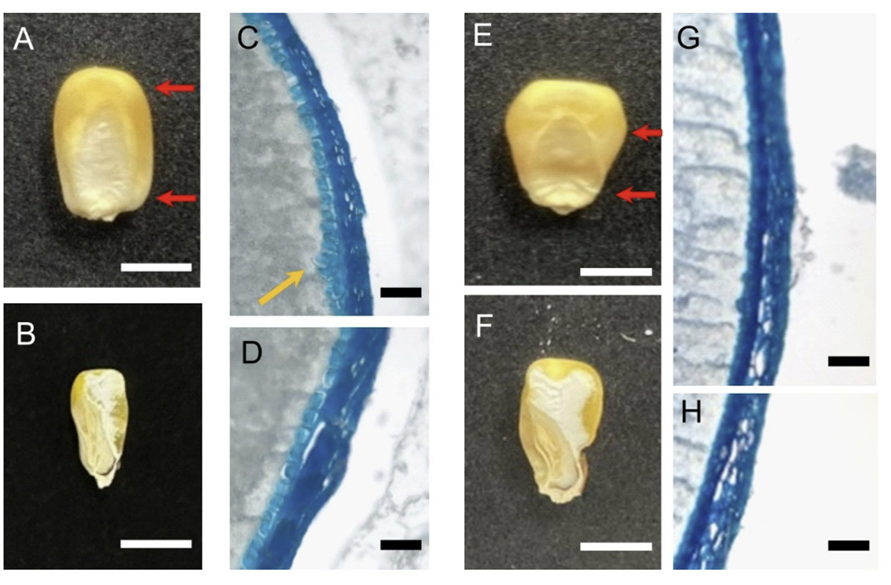
Observation of the distribution of aleurone layers in an entire cross section with the present method
We observed the overall appearance of aleurone layers in three maize genetic resources, thk1-R, Peru 442, and Coroico which exhibited characteristics of multiple aleurone layers using the presented method (Fig. 3). Upon examination of the overall aleurone layer, maize genetic resources with multiple aleurone layers exhibited irregular characteristics, suggesting the presence of varying layer numbers and arrangements. Specifically, the thk1 mutant exhibited the uniform formation of three or more layers in the aleurone (Fig. 3C and D), whereas in Coroico, the aleurone layers formed a singular layer with sporadic instances of irregular double layers in the upper region of the kernel (Fig. 3G and H). Similarly, Peru 442 displayed double layers as observed in Coroico (Fig. 3K), but in the lower part of the kernel, a single layer was observed (Fig. 3L). Based on these findings, the configuration of multiple aleurone layers varied depending on the position within a kernel. Considering the potential limitations of manual hand sections and the partial representation of the cross-sections, there was a risk of observing only fragmented portions of the maize aleurone layers, potentially leading to inaccuracies in assessing the layer count. However, this newly developed method offers a comprehensive perspective of the maize aleurone layers, ensuring accurate determination of the layer count. Consequently, we can effectively assess the aleurone layer characteristics of maize genetic resources and select suitable materials for breeding purposes, emphasizing the potential value of these genetic resources for future agricultural advancements.
Fig. 3
Phenotypes of maize genetic resources with multiple aleurone layers. (A) Kernel appearance of thk1-R, (B) longitudinal cross-section of a thk1-R kernel, (C, D) the aleurone layers of thk1-R sections observed by microscope, (E) the kernel appearance of the landrace Coroico, (F) longitudinal crosssection of a Coroico kernel, (G, H) the aleurone layers of Coroico sections stained with toluidine blue, observed under a microscope. (I) The kernel appearance of the landrace Peru 442, (J) longitudinal cross-section of a Peru 442 kernel, (K, L) the aleurone layers of Peru 442 sections stained with toluidine blue observed by microscope. Red arrows indicate points of microscope observation, the white scale bars represent 0.5 cm, and the black scale bars represent 50 μm. The yellow arrow indicates the double aleurone layer.
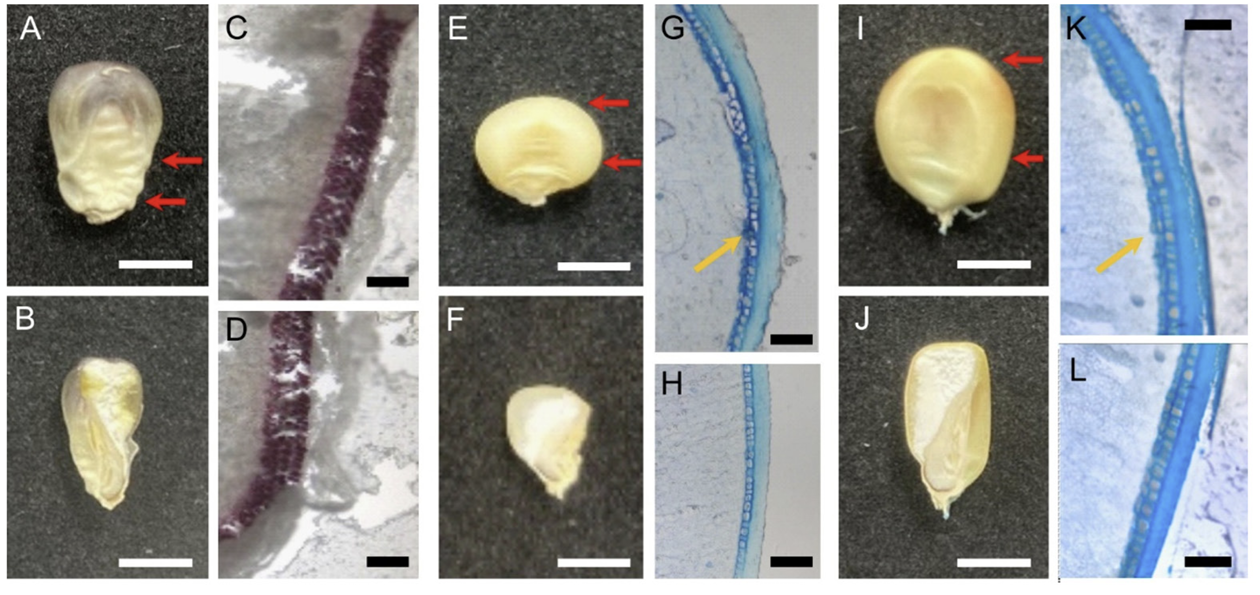
The first report of a Korean landrace having partial multi-aleurone layers
Using the presented method, here we report a novel maize landrace exhibiting characteristics of multiple aleurone layers. While Korean maize landraces are relatively well conserved for a long time since commercial cultivars were only recently introduced, research on them has been limited (Lim and Yi, 2019). The maize landrace collected from Ganghwa Island showed partial multiple aleurone layers (Fig. 4). While composed of a single layer in the upper part (Fig. 4C), irregular patterns of three or more aleurone layers were observed in the lower part of a kernel (Fig. 4E). The presence of maize landraces with multiple aleurone layers highlights their potential value for nutritional enhancement and advancement in the breeding of maize varieties. These results showed that there are certain levels of phenotypic and possible genetic diversity within Korean maize landraces and their possible implications for agricultural improvement.
Fig. 4
Seed and aleurone phenotypes in a Korean maize landrace collected from Ganghwa Island. (A) Kernel appearance of a Ganghwa Island landrace maize, (B) longitudinal cross-section of a Ganghwa Island maize kernel, (C, D, E) the aleurone layers of maize observed by microscope. Red arrows indicate points of microscope observation, the white scale bars represent 0.5 cm, and the black scale bars represent 50 μm. The yellow arrow indicates the multi-layer aleurone.
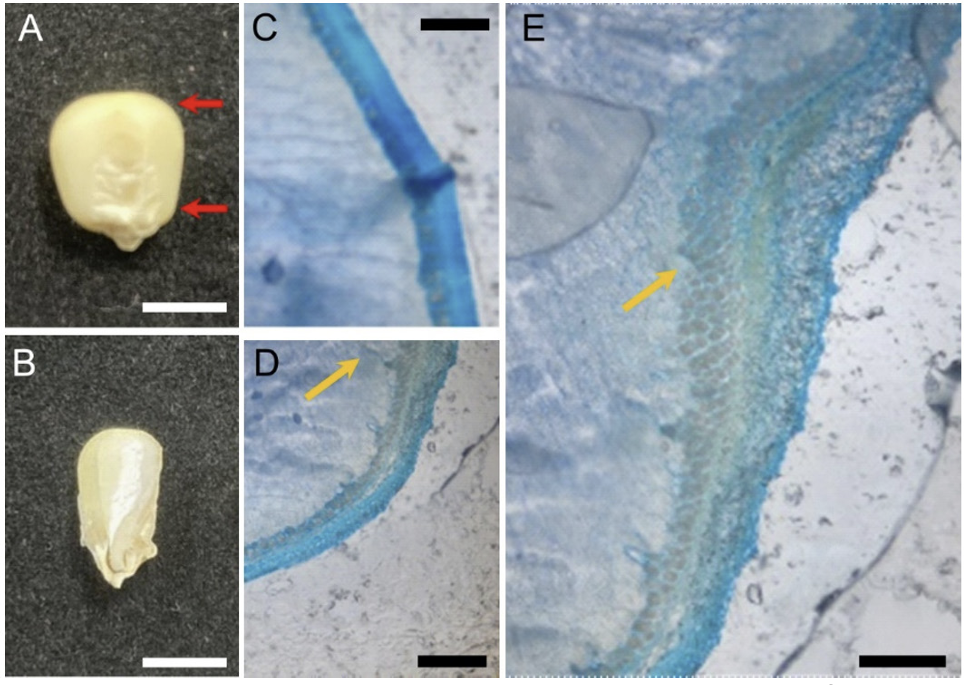
Conclusion
Our study has effectively streamlined the microscopy sample preparation process including paraffin embedding and microtome dissections for a comprehensive visualization of the aleurone layer across the entire cross-section in maize. The developed method notably reduced the time compared to conventional approaches. Entire cross-section observation enabled to identify the sporadic formation of the multi-aleurone layer in B73 and Coroico and the positional irregularity of the multialeurone phenotype in a Korean landrace. Therefore, it contributes to a better understanding of aleurone property in maize genetic resources and may have implications for agricultural and breeding advancements in the future.