Introduction
Phosphorus (P) is one of the major essential macronutrients for plants and is applied to soil in the form of chemical P fertilizer; however, the synthesis of chemical P fertilizer is a highly energy intensive process and has long term implications for the environment in terms of eutrophication, soil fertility depletion, destruction of micro-organisms, and carbon footprint (Sharma et al., 2013).
When applied to soil, P easily transforms into an insoluble and stable form with limited availability to plants and only less than 5% of the total amount of P in soil is available for plant nutrition (Pundarikakshudu, 1989; Boronin, 1998).Given the negative environmental impacts of chemical fertilizers and their increasing costs, the use of phosphate solubilizing microorganisms (PSM) is an advantageous sustainable agricultural practice (Harman, 1992).
Microorganisms that convert insoluble phosphates into soluble forms are termed to phosphate solubilizing microorganisms (PSMs). Microorganisms such as Alcaligenes sp., Aerobactor aerogenes, Achromobacter sp., Actinomadura oligospora, Burkholderia sp., Pseudomonas sp., Bacillus sp., and Rhizobium sp. are the most important phosphate solubilizers in soils. Apart from bacterial species, some fungal stains are also reported to be phosphate solubilizers such as Aspergillus sp. (A. awamori, A. niger, A. tereus, A. flavus, A. nidulans, A. foetidus, A. wentii.) and Penicillium sp. (P. digitatum, P. lilacinium, P. balaji, P. funicolosum).
The use of microbial inoculants (as biofertilizers) possessing P-solubilizing activity in agricultural soils is considered as an environmentally-friendly way which is an alternative to applications of chemical based P fertilizers (Zaidi et al., 2009). It has been reported that plant growth promoting rhizobacteria (PGPR) including (PSMs) are able to solubilize the unavailable forms of P in soil by acidification, chelation, ion exchange reactions, and production of low molecular weight organic acids such as gluconic, oxalic, and citric acids (Jones, 1998). The main mechanism of phosphate solubilization is the production of some organic acids. Among the organic acids produced, gluconic, formic, 2-ketogluconic, citric, oxalic, lactic, isovaleric, succinic, glycolic, and acetic acids can be produced from P- solubilizing bacteria. Production of these organic acids results in the lowering of pH in the surrounding. The lowering of pH of the medium suggests the release of organic acids by the P-solubilizing microorganisms via the direct oxidation pathway that occurs on the outer face of the cytoplasmic membrane (Whitelaw, 2000; Maliha et al., 2004). Many reports suggest a positive correlation between the lowering of pH andsoluble P concentration in the medium (Perez, 2007). In addition to providing available phosphorus to plants, phosphate solubilizing microorganisms can enhance plant growth through several different mechanisms, such as symbiotic nitrogen fixation, ammonia production, production of plant hormones, and control of phytopathogenic microorganisms (Rangarajan et al., 2003; Ali et al., 2010). Therefore, the use of microorganisms with higher phosphate solubilizing abilities has proved to be an economically sound alternative to the more expensive chemical fertilizers and thus possesses a greater agronomic utility. However, they are not present in enough quantitiesin the rhizosphere of plants. Thus, inoculation of plants by a target microorganism at a higher concentration than that normally found in soil is necessary to take advantage of the property of phosphate solubilization for plant yield enhancement (Vessey, 2003). Moreover, there are several previous reports suggesting that co-inoculation of two or more microbial strains having aphosphate solubilizing capacity stimulates plant growth and yields more than their separate inoculations (Chen et al., 2008).
Keeping this in view, the present study was planned to evaluate the effect of co-inoculation of phosphate solubilizing bacteria, B. cepacia and A. aquatilis, on solubilization of inorganic phosphate in the growth medium and their effect on growth and yield of maize plant under greenhouse and field conditions.
Materials and Methods
Sample site and collection
Maize rhizosphere soil samples were collected from eight different areas of Nainital region (29°23′N 79°27′E/ 29.38°N 79.45°E/ 29.38; 79.45) of Uttarakhand, India. Plants were dug out, and the excess bulk soil was removed by gentle shake. The soil adhering to the root was considered rhizosphere soil (Von der Weid et al., 2000) and collected in sterilized plastic bags.
Isolation of phosphate solubilizing bacteria
One gram (1g) of each soil sample was dispersed in 9 mL of autoclaved distilled water, filtered by 125 mm Whatman® qualitative filter paper, Grade 1. One mL of the above filtered solution was again transferred to 9 mL of sterile distilled water to form 10-2 dilution. Similarly, 10-3, 10-4, 10-5, 10-6, 10-7, and 10-8 serial dilutions were made for each soil sample (Hou et al., 2008). The diluted (10-4, 10-5, and 10-6) soil samples were plated (0.1 mL) on Pikovskaya’s (PVK) agarcontaining tricalcium phosphate (TCP) as the phosphate source (Pikovskaya, 1948). Pikovskaya’s medium consisted of yeast extract 0.50 g/L, dextrose 10.00 g/L, calcium phosphate 5.00 g/L, ammonium sulphate 0.50 g/L, potassium chloride 0.20 g/L, magnesium sulphate 0.10 g/L, manganese sulphate 0.0001 g/L, ferrous sulphate 0.0001 g/L, agar 15 g/L, and dissolved in 1,000 mL distilled water. The pH of the media was adjusted to 7.0 before autoclaving at 15 psi pressure (121℃) for 15 minutes. Following autoclaving, the media were well mixed and poured into sterile Petri plates (25 mL/plate) under a laminar flow hood, which was then allowed to solidify.
Inoculated plates were incubated in an inverted position in an incubator for up to 7 days at 27℃ - 30℃. Colonies with a clear halo were marked positive for phosphates solubilization and were considered as phosphate solubilizing bacteria (PSB). These selected colonies were again subcultured (2 - 3 times) on the same PVK media at 30℃ by the striking method until pure cultures of bacteria were obtained. Isolated bacteria were kept on PVK agar slants at 4℃ for further study.
Genomic DNA isolation, PCR amplification, and sequencing of 16S rDNA gene
Total genomic DNA of bacterial isolates was extracted by CTAB method. After DNA extraction, PCR reaction was performed in a VeritiThermal Cycler (Thermo Fisher Scientific). The universal primers (27F Forward primer 5'-AGAGTTTGATCCTGGCTCAG-3' and 939r reverse primer 5'-CTTGTGCGGGCCCCCGTCAATTC-3' were used to amplify about 1,000 bp fragment of 16S rRNA gene. PCR amplifications were carried out in 50µL PCR reaction mixture consisted of 3 mL template DNA (100 ng - 1.0 mg), 25 mL 2 × KAPA Taq ready mix [containing dNTPs (0.2 mM of each dNTP at 1 ×), MgCl2 (1.5 mM at 1 ×)], and 1µL of both forward and reverse primers. The amplification cycle consisted of an initial denaturation step of 3 min at 93℃, followed by 35 cycles of 30 sec. at 95℃ (denaturation), 30 sec. at 60℃ (annealing), and 30 sec. at 72℃ (extension), with a final extension step of 3 min at 72℃. PCR products were electrophoresed using 1.5% agarose, stained with ethidium bromide (0.5 µg/mL), and visualized using a Gel luminax 312. PCR products of isolates were purified using a PCR purification kit (Qiagen, Germany) according to the manufacturer’s instructions and the amplified products were sequenced on the Sanger sequencing platform at Pragti Biomedical in New Delhi. All the bacterial isolates were classified by BLAST analysis of their respective 16S rRNA gene partial sequences. Various databases including eztaxon, NCBI (National Center for Biotechnology Information), and Ribosomal Database Classifier (RDP) were used to determine the exact taxonomical classification of individual organisms (Hamid et al., 2010). For the determination of the closest type strains, NCBI BLAST was used (Zhang et al., 2000).
Phylogenetic analysis
Sequence data were compared visually. Sequences were aligned using the Clustal W software and their distances were calculated according to Kimura’s two-parameter method (Kimura, 1980). Phylogenetic trees were produced using the neighbour-joining method. Bootstrap analysis was based on 1,000 resamplings. The MEGA (Molecular Evolutionary Genetics analysis) 6.05 package was used for all phylogenetic analysis (Tamura et al., 2013). The final sequence was submitted to GenBank (Thompson et al., 1997).
Inoculum preparation
Single colonies were each transferred into 100 mL flasks containing 25 mL nutrient broth and grown aerobically in flasks on an orbital shaker at 160 rpm for 48 hr at 30℃. Bacterial cells were then harvested by centrifugation for 5 min at 13,081 × g (10,000 rpm), 21℃, in RAMI centrifuge, and washed with sterile distilled water. The cell pellet was resuspended with sterile distilled water, and then cells were to 2.0 × 108 colony forming units (CFU)/mL (at O.D 430 nm). Sterilized uninoculated medium served as a control. The supernatant was used to determine phosphate solubilization index, the level of phosphorous release, pH, and organic acid production.
Analysis of phosphate solubilizing activity
The qualitative and quantitative analyses of phosphate solubilizing activity of the selected isolates were conducted by the plate screening method and broth culture method, respectively.
Qualitative measurement of phosphate solubilization
Bacterial isolates were screened for their TCP solubilizing activity on PKV plates. Isolates were spot inoculated on the center of agar plate aseptically. The plates were incubated at 28 ± 2℃ for 5 days. A clear zone around a growing colony indicated phosphate solubilization and was measured as phosphate solubilization index (SI). SI was calculated as the ratio of the total diameter (colony + halo zone) to the colony diameter (Edi-Premono et al., 1996).
xmlns:xlink="http://www.w3.org/1999/xlink" xlink:href="http://dam.zipot.com:8080/sites/kjoas/images/N0030440204_image/PICE588.gif" />
All observations were recorded in triplicate. Strains developing clear zones around their colonies could easily be identified as PSBs.
Quantitative measurement of phosphate solubilization and pH of the media
Quantitative estimation of solubilized P by bacterial isolate was done by the vanadomolybdophosphoric yellow colour method (Subba Rao et al., 1982) in Pikovskaya’s broth containing 5,000 µg/mL tri calcium phosphate.
The phosphate solubilizing ability of each isolate was tested by using insoluble tricalcium phosphate [Ca3(PO4)2] as sole P source in Pikovskaya’s medium. Ten mL of Pikovskaya’s broth containing 5,000 µg/mL P in the form of tri calcium phosphate was inoculated with 0.1 mL of bacterial culture (inoculum adjusted to - 2 × 108 CFU/mL) and incubated at 28℃ ± 2 for upto 8 days. One mL of the filtered supernatant (filter with 0.45 µM milliporefilter) was taken out on the 2nd, 4th, 6th, and 8th days after incubation. The supernatant was obtained by centrifugation at 10,000 rpm for 20 min and passed through a 0.45 µM millipore filter. An aliquot (0.1 mL) of the filtered supernatant was then mixed with 0.25 mL of Barton’s reagent and the volume was made up to 5 mL with double distilled water. After 10 minutes, the intensity of the yellow color was read using aspectrophotometer (UV-VIS Spectrophotometer-SL-159, Elico, India) at 430 nm and the amount of P-solubilized was extrapolated from the standard curve. The experiments were conducted in triplicates and values were expressed as their mean.
Pot experiment
A pot experiment was carried out in a greenhouse during May-June 2016 with four replications. It consisted of one control (non-inoculated with PSB) and three PSB species. The test was conducted in pots (5 cm in diameter) containing 500 mg of soil making a depth of 15 cm. The soil came from an agricultural field of maize of Haldwani, Uttarakhand. The unsterile soil (silt loam, pH 7.5 - 8.0 mg/100 g; total organic C, 0.10%; available N, 36.8 mg/100 g; available P, 5 mg/100 g; available K, 12 mg/100 g; total Ca, 39.816 mg/100 g; total Na 22 mg/100 g; total Mg, 40.1 mg/100 g) was thoroughly mixed, passed through a 2 mm sieve, and dried in sunlight for 7 days before being sterilized three times by dry heat treatment for 2 h at 121℃. Tricalcium phosphate (TCP) was supplied as soil P fertilizer at the rate of 160 mg kg-1 based on the nutrient requirements of maize.
For testing the efficiency of isolated phosphate solubilizing bacteria, its effect was observed on the growth of maize plants in vitro. Seeds were surface sterilized with sodium hypochlorite (2%) and alcohol (70%) for 1 min. Then seeds were washed with autoclaved distilled H2O to remove the small amount of sterilization chemicals. The broth culture for each of the isolates (single colonies) was made in Pikovskaya’s liquid medium. For this purpose, PSB suspensions were prepared by culturing the bacterial isolates (single colonies) on Pikovskaya’s media and incubating in 50 mL conical flask and placed it on orbital shaker at 160 rpm for 48 hr at 30℃. Then, bacterial cells were harvested by centrifugation for 5 min at 13,081 × g (10,000 rpm), at 21℃, in RAMI centrifuge, and washed with sterile distilled water. The cell pellet was resuspended with sterile distilled water, and cells were adjusted to 2.0 × 108 colony forming units (CFU)/mL (at O.D 430 nm). After making the broth culture, an equal number of seeds of maize were soaked in each broth culture separately for 12 hr at 28℃. Seeds were potted (4 per pot) in the sterile soil. The previously prepared 2 mL of broth culture were uniformly applied onto seeds which were then covered uniformlywith a 3-cm layer of soil. One pot was kept as control in which the seeds were potted in sterile soil without soaking in any culture of phosphate solubilizing bacteria. Pots were watered daily to maintain the soil field capacity during the study period. Agronomic variables (shoot and root length and dry weigh) were evaluated 20 days after inoculation. At the end of the study, plant growth parameters - such as % seed germination, shoot length, root length, shoot dry matter, root dry matter, and number of leaves were calculated.
Percent seed germination was calculated by the following formula:
xmlns:xlink="http://www.w3.org/1999/xlink" xlink:href="http://dam.zipot.com:8080/sites/kjoas/images/N0030440204_image/PICE598.gif" />
Field trial
To determine the productivity of maize, a field study on maize was carried out after the pot experiment. All potted plants were dug out and placed into field soil. The field trial was conducted on same soil used for the pot study. The experiment was conducted in Haldwani, Uttarakhand (29.2183°N, 79.5130°E), in the period of June to September 2016. There were four rows in each plot in which the row width and length were 0.7 and 5 m, respectively. Data regarding yield parameters was recorded at maturity in September 2016 (120 days after sowing). Before starting the field trial, the soil was fertilized with N, P, and K at rates of 300, 160, and 50kg ha-1 as urea, phosphate, and potassium sulphate, respectively,according to the nutrient requirements formaize. No other conventional fertilizers were used during the experiment.
Statistical analysis
Three or four replicates were used for each experiment. Data were analyzed by analysis of variance (ANOVA) and the means were compared with Tukey’s test at p < 0.05. In the field experiment, the plots with different treatments were arranged in a randomized complete block design with three replicates per treatment.
Results
Isolation and Identification of Phosphate Solubilizing Bacteria (PSB)
In the present study, the collected soil samples were evaluated in vitro for P solubilizing bacteria in Pikovskaya’s (PKV) plates supplemented with 1.5% (w/v) agar. A total of 8 phosphate solubilizing bacterial strainswere isolated on PVK agar medium, containing tri-calcium phosphate (TCP). Out of 8 bacterial isolates, 2 isolates (C1 and H6) were found to be potent phosphate solubilizers showing clear halo zones around theircolonies (Table 1).The halo zone formation around the bacterial colonies could be due to the production of organic acids, polysaccharides, or to the activity of phosphatase enzymes of phosphate solubilizing bacterial strains (Halder et al., 1993; Goenadi et al., 2000; Paul and Sinha, 2013a, 2013b).
Table 1. Identification of the most promising bacterial isolates based on their sequencing results and BLAST analysis.
![]() |
Genomic DNA isolation, PCR amplification and sequencing of 16S rDNA gene
The extracted DNA was analyzed byagarose gel electrophoresis. Good quality intact DNA with high molecular weight was obtained from C1 and H6 isolates. The genomic DNA of the twoisolates was amplified by 16S rRNA and was run on agarose gel (1.5%). The isolates indicated apositive signal in the form of discrete and distinct 1,000 bp bands on gel (Fig. 1).
Genotypic identification and phylogenetic analysis
The BLAST search performed against GenBank revealed a large number of similar 16S rRNA gene sequences. The BLAST results of most promising bacterial isolates showed > 99% similarities between available GenBank entries in which C1 isolate was identified as Burkholderia cepacia (KY435830) and H6 was identified as Alcaligenes aquatilis (KX345927) (Table 1). These sequences were submitted to the NCBI database and the accession numbers were obtained. Fig. 2 showes a phylogenetic tree including the isolates (C1 and H6) from this study and some closely related sequences obtained from NCBI. Two distant phylogenetic groups corresponding to the genera Burkholderia sp. and Alcaligenes sp. were obtained. In the phylogenetic group of genus Burkholderia, the isolate C1 was closely related to B. cepacia and H6 isolate was closely related to A. aquatilis. The number beside the node is the statistical bootstrap value. In brackets are the GenBank accession numbers of the 16S rRNA genes.The tree was rooted using Enterobacter cloacae ATCC13047 (AJ251469) as outgroup.
Fig. 2.
Phylogenetic tree based on 16S rDNA gene sequences showing the position of Burkholderia cepacia (C1) and Alcaligenes aquatilis (H6) strains to related species, which was generated based on pairwise nucleotide distance of the Kimura 2-parameter using the neighbor-joining method included in the MEGA 6.05 software package. The scale bar indicates 0.02 substitutions per nucleotide position. The number beside the node is the statistical bootstrap value. In brackets are the GenBank accession numbers of the16S rRNA genes.
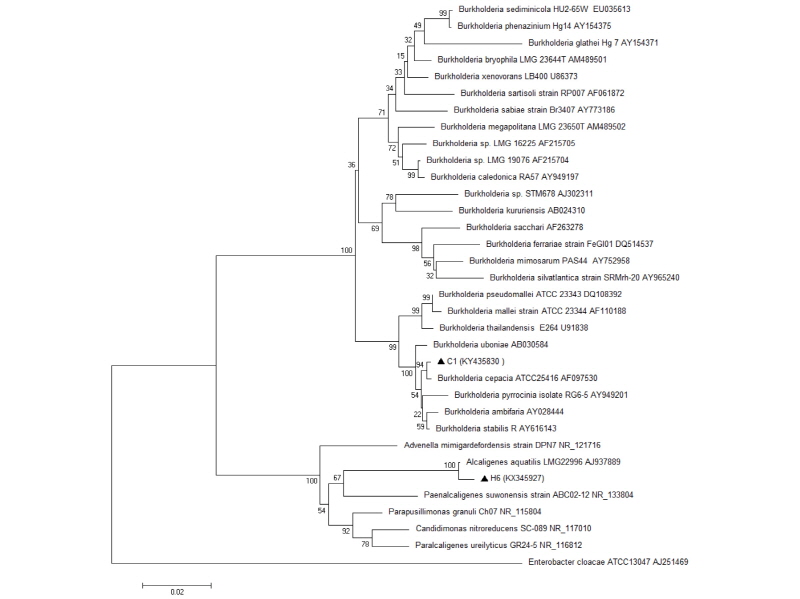
Analysis of phosphate solubilizing activity
Qualitative measurement of phosphate solubilization
Qualitative P-solubilization potential was anticipated by observing the large clear halo zones around the bacterial colonies on Pikovskaya agar media, and phosphate solubilization index (PSI) was calculated. Two isolates were found to have ≥ 4.5PSI. C1 isolate showed 4.88 PSI index followed by H6 (4.64) (Fig. 3). PSI of co-inoculation of two strains was 5.25.
Quantitative measurement of phosphate solubilization
As showed in Fig. 4, in medium containing Ca3(PO4)2,solubilization is slow in the first two days of incubation and then becomes fast, reaching the highest level (309.66 µg/mL) on the 8th day after whichno further change in soluble P concentration was observed (data not presented). The amount of P-solubilized was extrapolated from the standard curve (Fig. 5). Maximum P solubilization was observed with C1 (305.49 µg/mL) followed by H6 (282.38 µg/mL). Co-inoculation of two strains was found to release the highest content of soluble phosphorus (309.66 µg/mL) into the medium. It was also found that the growth of the isolate caused a significant increase of acidity in the medium containing Ca3(PO4)2.On the 8th day, the pH of the culture medium declined to 3.08 ± 0.08 from the initial pH of 7.11 (Fig. 6). After the 8th day, no further pH change was seen (data not presented). Minimum pH was observed with C1 (3.08) followed by H6 (3.82). In co-inoculation, the highest pH (3.02) reduction was observed in the culture medium.
Pot examination
For determination of plant parameters, all maize plants were harvested after 20 days of inoculation and the growth parameters, such as shoot and root height, fresh and dry weight, number of leaves, and leaf lengths were recorded. The shoots were separated from the roots at 0.5 cm above soil surface. The roots were washed out with tap water to remove soil particles. Dry weight of roots and shoots were measured after drying in an oven for 48 hr at 60℃ and compared with control plant. Plants inoculated with C1 isolates showed shoot height of 6.5 cm, shoot fresh weight of 1,060 mg, shoot dry weight of 480 mg, root length of 13.875 cm, root fresh weight of 610 mg, and root dry weight of 520 mg, and leaf height 13.07 cm. Plants inoculated with H6 isolates showed average shoot height of 6.15 cm, shoot fresh weight of 1,040 mg, shoot dry weight of 390 mg, root length of 13.27 cm, root fresh weight of 540 mg,and root dry weight of 260 mg, and leaf length of 12.35 cm (Table 2). Furthermore, co-inoculated maize seedlings showed shoot height of 5.95 cm, shoot fresh weight of 1,120 mg, shoot dry weight of 370 mg, root height of 11.65 cm, root fresh weight of 500 mg, and root dry weight of 450 mg, and leaf length of 10.62 cm.
For plants co- inoculated with the two isolates, we found that there was significant deference (p < 0.05) in their growth between co-inoculated plant and control plant. Based on the plant growth bioassay, co-inoculated maize seedlings showed significantly increased shoot heights (75%), shoot fresh weights (93.10%), shoot dry weights (84.99%), maximum root lengths (55.95%), root fresh weights (66.66%), root dry weights (275%), and maximum leaf lengths (81.53%), compared to the control. However, there was no significant difference between plants inoculated with a single bacterial strain (C1 and H6) and co-inoculated with both bacterial strains in phosphate solubilization index, phosphorous release, pH of the media, and plant growth parameters.
Field trial
In a field trial, plants inoculated with the two isolates (C1 and H6) showed significant increases in cob length, number of grain per cob, and grain weight. Plants inoculated with C1 isolates had cob length of 19.93 cm, 383 grains per cob, and 42.5 g/100-grain and plants inoculated with H6 isolates showed cob length of 17.3 cm, 367 grains per cob, and 39.83 g/100-grain. However, co-inoculated plants showed significant increase as the plants had cob length of 21.56 cm (136.92%), 331 grains per cob (46.68%), and 41.43 g/100-grain (67.46%) over control (Table 3).
Discussion
The use of efficient PSM opens a new window for the better crop growth and productivity with sustainable soil health. However, the viability and sustainability of PSM technology largely depend on the development and distribution of good quality microbial inoculants to farming communities. Therefore, we need to identify and characterize more PSM with high efficiency for their application under various field conditions. The use of PSM in agricultural soils is a low-cost technology and environmentally-friendly approach which doesn’t disturb ecological balances (Das et al., 2007). Studies in liquid cultures revealed that PSM increased available phosphorus by solubilizing suspended tricalcium phosphate (TCP) due to the release of organic acids into the surrounding medium (Gaur, 1990). Previous reports also described some Burkholderia, especially a B. cepacia strain, as being efficient phosphate solubilizers (Rodrıguez et al., 1999; Hariprasad et al., 2009; Khalimi et al., 2012).
Phosphate solubilization potential has been attributed to the strain’s ability to reduce pH of the surroundings either by releasing organic acids (such as gluconic acid, formic acid, oxalic acid, and citric acid) or protons (Chen et al., 2006). In the present study, the isolates were identified using the modern 16S rRNA technique. According to the sequence of the 16S rRNA gene, C1 isolates belong to B. cepacia (99% sequence homology) and H6 isolates belong to A. aquatilis (99% sequence homology) according to NCBI database (Table 1). Fig.2 shows a phylogenetic tree including the isolates (C1 and H6) from this study and some closely related sequences obtained from NCBI. Two distant phylogenetic groups corresponding to the genera Burkholderia sp. and Alcaligenes sp. were obtained. In the phylogenetic group of genus Burkholderia, the isolate C1 was closely related to B. cepacia and the isolate H6 was closely related to A. aquatilis. The number beside the node is the statistical bootstrap value. In brackets are the GenBank accession numbers of the 16S rRNA genes.The tree was rooted using Enterobacter cloacae ATCC13047 (AJ251469) as outgroup.
Moreover, two efficient PSB strains (B. cepacia and A. aquatilis), which had a marked insoluble phosphate solubilizing ability, were isolated. We found that the phosphate solubilization index for the co-inoculation of two strains was 5.25, followed by 4.88 for single inoculation of C1 and 4.64 for H6. Co-inoculation of two strains was found to release the highest content of soluble phosphorus (309.66 µg/mL) into the medium, followed by single inoculation of C1 (305.49 µg/mL) and H6 (282.38 µg/mL). In addition, co-inoculation, the greatest reduction of pH (3.02) was observed in the culture medium inoculated with C1 (pH 3.08) and H6 (pH 3.82) strains compared to that of single inoculation. We also found that the soluble P concentration was inversely correlated to the pH of the culture medium during the growth period of bacteria. This was reported earlier by Chen et al. (2006) and Xiang et al. (2011). The data showed a negative correlation (r = -0.68) between pH and soluble P content of the medium and apositive correlation (r = 0.96) between soluble P content and PSI, suggesting that acidification of the medium can facilitate phosphate solubilization. The negative correlation between the pH and soluble phosphorous content of the medium, as well as the positive correlation between soluble phosphorous content and solubilization index, suggested that acidification of the medium could facilitate phosphate solubilization.
However, our results contrasted with the report of Tao et al. (2008), which documented that there was no correlation between pH of culture medium and P mineralization by the organic phosphorus mineralizing bacteria, suggesting that mineralization of organic phosphorus may have different mechanisms of phosphorus solubilization.Comparatively, co-inoculation showed higher phosphate solubilizing enhancement than single inoculation, suggesting that both strains acted synergistically in phosphate solubilization. Yu et al. (2011) also reported similar results after inoculation of Pseudomonas chlororaphis and Bacillus megaterium.
We found a significant difference (p < 0.05) in growth between co-inoculated plants and control plants. Based on the plant growth bioassay, co-inoculated maize seedlings recorded significantly increase in shoot height (75%), shoot fresh weight (93.10%), shoot dry weight (84.99%), root maximum length (55.95%), root fresh weight (66.66%), root dry weight (275%), maximum leaf length (81.53%), compared to the control. However, there was no significant difference, between the plants that were single inoculated with bacterial strain (either with C1 orH6) and plants that were co-inoculated with these two bacterial strains, in phosphate solubilization index, phosphorous release, pH of the media, and plant growth parameters. In addition, phosphate solubilization is not the only way PSBs promotethe growth of plants, they also facilitate the growth of plants by stimulating the production efficiency of plant hormones such as auxins, cytokinins, gibberellins, and some volatile compounds, (Podile et al., 2006). Therefore, enhanced plant growth after inoculation with PSB strains may be attributed to the ability of strains to make phosphorous available and to produce plant growth promoting substances simultaneously (Khalid et al., 2004; Linu et al., 2009; Li et al., 2010).
Both strains used in this study exhibited the capacity to produce gluconic acid (C1), formic acid (C1), citric acid (H6) and unknown acid (C1) (data not shown), and this might have contributed to insoluble phosphates to solubilizing form (Chung et al., 2005). The present study’s findings correspond to the results reported by Afzal and Bano (2008) who observed that wheat (Triticum aestivum) inoculated with PSB (Pseudomonas sp. strain 54RB) had significantly increased root and shoot weights, plant height, spike length, grain yield, and P uptake compared to those of the control. Similar results were also found in cowpea (Vigna unguiculata) which revealed the enhancement of nodulation, root and shoot biomass, straw and grain yield, and P and N uptake of plants inoculated with Gluconacetobacter sp. and Burkholderia sp. (Linu et al., 2009).
Based on the results found in the present study, we conclude that A. aquatilis may be considered as a novel phosphate solubilizer. In addition, the B. cepacia strain has great potential for use as a soil inoculant when compared to other isolates. These PSB isolates hold good prospects for sustainable agricultural practices with minimal chemical inputs or for organic farming.In conclusion, this study revealed that co-inoculation of two stains gives greater enhancement of phosphate solubilization and plant growth parameters over the uninoculated control. However, there was no significant difference between the effects of single inoculation and co-inoculation ofthese bacterial strains, suggesting thatsingle inoculation or co-inoculation with B. cepacia and A. aquatilis could be used as an environment-safe biofertilizer for maize production.
Conclusion
This study has provided evidence that two bacterial strains (B. cepacia C1 and A. aquatilis H6) have significant effects on both phosphorus solubilization and plant growth enhancement by lowering pH along with the production of organic acids. Co-inoculation of two PSB strains acted synergistically and was responsible for the increase of several growth parameters in comparison with single inoculation. However, before using microbial isolates as a biofertilizer, their impact on crop productivity and quality should be investigated to confirm the present results.