Introduction
골격근은 수축속도와 에너지 대사의 형태에 따라 다양한 종류의 근섬유로 이루어져 있는데, 특히 골격근 내 주요 구조단백질인 myosin heavy chain (MyHC)의 종류에 따라 근육의 수축양상 및 에너지 대사가 크게 영향을 받는 것으로 알려져 있다(Pette and Staron, 2000). 다양한 유전자로 구성된 MyHC은 구성요소에 따라 I, 2a, 2x, 2b type으로 나뉘고, 수축형태는 MyHC I이 가장 느리게, MyHC 2b가 가장 빠르게 수축한다(Schiaffino and Reggiani, 1994). 근수축의 속도양상에 따라 골격근의 에너지 대사가 바뀌게 되는데, 빠르게 수축하는 근육에는 에너지를 빨리
만들어낼 수 있는 glycolytic metabolism이, 느리게 수축하는 근육에는 oxidative metabolism이 우점하는 것으로 알려져 있다(Matsakas and Patel, 2009). 따라서, 느리게 수축하는 골격근에는 mitochondria가 많고, 오랫동안 움직임을 유지할 수 있고, type 2 근육은 수축은 빠르게 할 수 있으나 쉽게 지치는 경향이 있다(Lim et al., 2016; Liu et al., 2016; Ryu et al., 2016; Scheffler et al., 2016; Toyama et al., 2016; Westerblad et al., 2010).
동물의 몸을 지탱함과 동시에 움직임을 관장하는 조직인 골격근을 구성하고 있는 근섬유는 에너지 대사와 수축속도 등의 환경요인에 따라 그 속성이 바뀔 수 있다(Matsakas and Patel, 2009). 근육수축이 지속될 경우, 특히 운동의 종류에 따라 골격근의 종류가 바뀔 수 있다. 예를 들어 싸이클 선수의 근육에서는 MyHC I 이 우점하고, 단거리 육상선수의 근육에서는 slow-twitch에서 fast-twitch 근섬유로 전환되는 현상이 보고된바 있다(Jansson et al., 1990). 하지만, 이러한 근육형질의 전환(transition)은 근수축의 형태 또는 에너지대사와 관련이 있다는 매우 극소수의 연구결과가 있을 뿐이고, 이에 대한 정확한 메커니즘은 아직 밝혀진 바가 없다. 따라서, 본 연구에서는 mitochondria의 산화적 인산화 과정을 저해하는 antimycin A를 이용하여 근육세포의 에너지대사 조절을 통해 근육의 형태가 전환되는지를 알아보기 위하여 수행되었다.
Materials and Methods
Cell culture
본 연구에 사용된 근육세포는 마우스 유래 세포주인 C2C12이고, 한국세포주은행(KCLB; Korean Cell Line Bank)에서 제공받았다. 세포는 Dulbecco’s modified Eagles medium (DMEM; Gibco BRL, USA)에 10% fetal bovine serum (FBS; Gibco BRL, USA), 10,000 U/mL penicillin, 10,000 µg/mL streptomycin (PS; Gibco BRL, USA), Fungizone (Gibco BRL, USA)을 함유하는 성장배지에 20,000 cells/cm2으로 희석하여 6 well plate에 분주하고 37℃, 5% CO2에서 배양하였다. 배지는 48시간 마다 교체해 주었다. C2C12 세포의 분화는 DMEM에 2% horse serum이 첨가된 배지를 이용하여 유도하였다. 세포가 분화되면 antimycin A (Sigma-Aldrich, USA)를 농도별로(0, 100, 200, 500, 700, 1,000 ng/mL) 72시간 동안 처리하였다.
Cell staining
분화율 분석을 위해 세포는 PBS로 washing 후 4% paraformaldehyde로 약 10분간 고정한 뒤, Giemsa (Invitrogen, USA)로 10분간 염색 하였다. 총 nuclei와 myotube 안에 있는 nuclei의 개수는 ImageJ (National Institute of Health, USA) 프로그램을 이용하여 분석하였다. 3개 이상의 muclei가 있는 세포를 myotube로 인정하였다. Fusion index는 총 nuclei 숫자에 myotube 안에 있는 nuclei 수의 비율로 계산하였다.
Western blot analysis
배양세포를 PBS로 세척한 후 lysis buffer (Sigma, USA)를 이용하여 용해한 후 13000 rpm에서 20분간 원심분리하여 상층액 부분의 단백질을 취하였다. 단백질은 BCA protein assay kit (Peirce, USA)을 이용하여 농도를 측정한 다음 동일한 양의 단백질을 취하여 실험에 사용 하였다. 100℃에서 5분간 denaturation 한 뒤 SDS-PAGE로 단백질은 분리하고, polyvinylidene difluoride (PDVF) membrane (Bio-Rad, CA)으로 옮긴 후 1시간 동안 상온에서 blocking buffer (5% skim milk + 0.1% tween 20 in PBS)로 배양하였다. Membrane은 anti-MyHC I (anti-slow, A4.840; Developmental Studies Hybridoma Bank, DSHB, USA) antibody, anti-MyHC 2b (anti-IIb, BF-F3; DSHB, USA) antibody, anti-MyHC all (BF-35; DSHB, USA)의 일차항체로 각각 상온에서 1시간 반응시켰다. 반응 후 membrane은 PBS로 3회 세척한 다음 상온에서 1시간 동안 horseradish peroxidase-conjugated secondary antibody와 반응시킨 후 ECL kit (Amersham Pharmacia, Sweden)을 사용하여 이미지화 하고, 밴드는 ImageJ 프로그램(Natinal Institute of Health, USA)을 이용하여 분석하였다.
Enzyme activity
Lactate dehydrogenase (LDH)의 활성은 1.5 mM pyruvic acid와 0.14 mM NADH, 0.1 M potassium phosphate buffer (pH 6.85)를 이용하여 NADH로부터 NAD+로 산화되는 정도를 spectrophotometer (UV-160, Shimadzu, Japan)로 340 nm, 25℃에서 측정하였다. 활성의 단위는 1분 동안 감소되는 흡광도 1.0을 1unit으로 계산 하였다. Creatine kinase (CK) 활성은 QuntiChrom creatine kinase assay kit (ECPK-100, BioAssay Systems, USA)를 이용하여 측정하였다. 세포를 assay buffer로 용해 한 뒤 enzyme mix와 혼합하여 96 well plate에 넣고 상온에서 10분간 정치 시킨 뒤 spectrophotometer를 이용하여 340 nm에서 30분 간격으로 O.D.를 측정하였다. Creatine kinase activity 계산식은 다음과 같다.
[CK (U/L) = O.D. 40 min – O.D.10 min / O.D.cal – O.D.H2O X 100]
흡광도 측정 후 샘플과 calibrator와의 백분율로 CK를 계산하였다. 1 U (unit)은 pH 6.0의 조건에서 phosphocreatine으로 부터 1 µmole의 phosphate가 ADP로 binding하여 ATP로 전환되는데 필요한 CK의 분당 활성도를 나타낸다.
Statistics
본 시험결과의 통계분석은 통계분석프로그램 R3.3.2를 이용하여 분산분석(ANOVA)을 실시하여 5% 수준에서 유의성을 검정하고, Tukey HSD test를 이용하여 사후검정하였다.
Results and Discussion
Muscle cell morphology
본 연구에서는 mitochondria의 전자전달계를 억제하는 antimycin A를 근육세포에 처리 한 후, 근육세포 내 에너지 대사를 변화시킴에 따른 근육구조단백질인 myosin heavy chain (MyHC)의 발현과 관련 효소의 활성에 미치는 영향을 분석하였다. Antimycin A를 cancer 세포에 처리하여 apoptosis를 유도하였던 이전 연구결과(Han et al., 2008)에서는 높은 농도의 antimycin A (2 ~ 100 µM)이 사용되었지만 본 연구에서는 mitochondria의 산화적 인산화를 억제할 수 있는 최소한의 농도(~1.8 µM)를 사용하여 세포의 apoptosis는 관찰되지 않았다. 또한, antimycin A 처리가 근육세포의 분화에 미치는 영향을 분석하기 위하여 Giemsa staining을 실시한 결과, Fig. 1에서와 같이 대조구와 각 처리구별 분화정도(fusion index)에는 차이가 없는 것으로 나타났다. 결과에서는 제시하지 않았지만 분화된 근육세포의 myotube 성상(숫자, 길이 및 직경)에도 처리구간 차이가 없었다. 근섬유의 숫자와 크기가 에너지대사에 영향은 받는 것은 당연하지만 antimycin A의 처리농도가 낮아서 세포의 수나 크기에는 영향이 없었을 것이라 사료된다. 에너지 대사관련 AMP-activated protein kinase (AMPK) 신호전달체계가 mitochondrial biogenesis나 fission에 영향을 준다는 결과(Toyama et al., 2016)를 토대로, 향후 근육세포 내 에너지 대사와 mitochondrial DNA 및 관련 신호전달체계에 대한 연구도 진행할 예정이다.
Fig. 1.
Effect of administration of antimycin A on differentiation of C2C12 myoblasts. (a) Representative Giemsa staining images of C2C12 myotubes treated with different concentration of antimycin A (0, 100, 200, 500, 700, 1,000 ng/mL). (b) Fusion index at 72 h of differentiation. Fusion index was calculated as the percentage of total nuclei that resided in cells containing 3 or more nuclei.
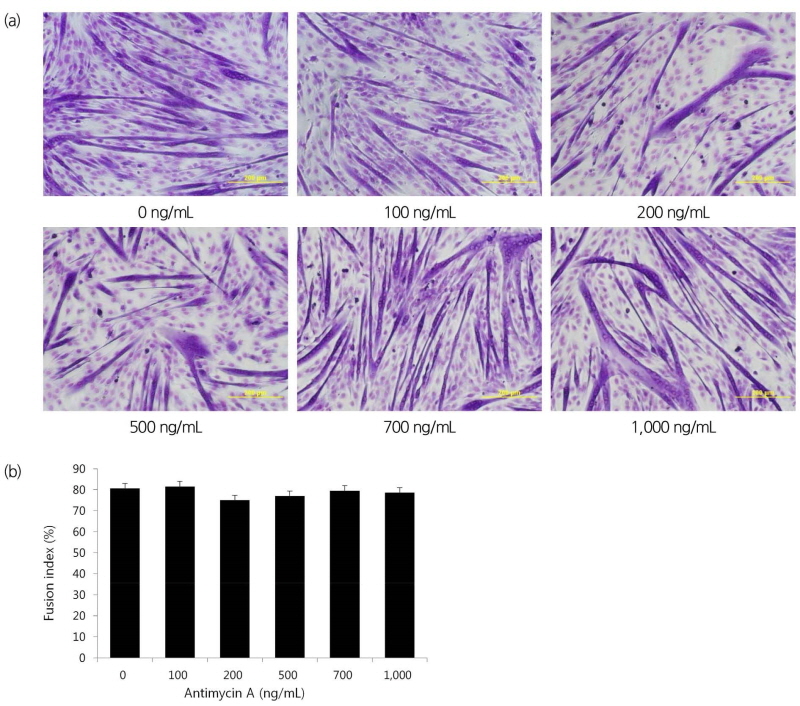
Myosin heavy chain expression
본 연구의 주요 가설인 mitochondrial activity 저하에 따른 골격근의 발현 성상이 slow-twitching type에서 fast- twitching type으로 변화될 가능성을 확인하기 위하여 근육의 구조단백질 발현양상을 분석하였다. 가설에서 예상한 데로 antimycin A를 처리한 근육세포의 myotube에서는 MyHC isoform 중에서 type 2b가 antimycin A 농도가 증가함에 따라 증가하여 농도 500 ng/mL이상에서 유의적으로 증가하였다(p < 0.05; Fig. 2). 골격근의 유전프로그램은 매우 세밀하게 조절되는데, mitochondia의 숫자와 bioactivity를 비롯한 에너지 대사와 대표적인 구조단백질인 MyHC의 발현양상은 서로 상호적으로 연관되어 있는 것으로 알려져 있다(Handschin et al., 2007). 이론적으로는 aerobic capacity가 높은 slow-twitching 근육에서MyHC type I의 발현과 더불어 mitochondria의 숫자가 가장 많고, fast-twitching 근육에서는 반대로 MyHC 2b의 발현이 높게 나타난다(Seifert et al., 2012). 이러한 에너지 대사 profile과 MyHC 발현 양상의 관계에서 볼 때, antimycin A 처리에 따른 aerobic metabolism 저해로 인해 aerobic metabolism을 주로 사용하는 MyHC slow의 발현이 줄어드는 패턴을 보이다가 1,000 ng/mL에서 유의적으로 줄어들고(p < 0.05; Fig. 2), MyHC 2b의 발현이 증가하였을 것으로 사료된다. 골격근의 특징 중에서 지속적인 외부자극에 대한 적응능력(adaptation)이 있는데, 이는 외부자극에 대응하기 위해 골격근 내 기능적인 변화를 유도하기 위해 근육의 형태를 바꾸는 것을 뜻한다(Handschin et al., 2007). 이러한 근육적응(adaptation) 또는 형질전환(transition)의 패턴은 I ↔ 2a ↔ 2x ↔ 2b 의 순서로 나타난다(Pette and Staron, 2000). 이번 연구의 결과에서는 MyHC I과 MyHC 2b만 분석하였는데, 이는 아직 MyHC 2x에 대한 항체의 역가에 대해 논박이 많은 상태이기 때문이다(Gunawan et al., 2007). 또한, MyHC I과 MyHC 2b mRNA와 protein 발현 간에 아주 높은 상관관계가 밝혀짐(Schiaffino and Reggiani, 1996)에 따라, MyHC I과 MyHC 2b의 발현 차이를 측정함으로써 근육의 형질전환 분석이 가능할 것으로 사료 되었다. 하지만 이번 연구의 결과에서 MyHC 2b의 발현정도가 antimycin A 처리 500, 700, 1,000 ng/mL에서 대조구에 비해 유의적으로 증가함에 비해, MyHC slow의 발현은 1,000 ng/mL에서야 유의적으로 감소되는 것으로 나타났다(p < 0.05; Fig. 2). 이는Western blot 기법을 이용한 MyHC의 발현 분석에서 type I과 type 2b antibody의 sensitivity의 차이일 수도 있고, 실험 과정상의 오차일수도 있는데, 이러한 분석기법에서의 차이 외에도 유전자 및 단백질 발현과정에서 MyHC2a 또는 유사 MyHC의 발현에 의해 이러한 차이점이 유래 되었을 가능성도 배제할 수 없을 것이다. 근육의 구조단백질인 MyHC의 발현 변화는 앞서 기술한 바와 같이 shifting의 개념으로 어느 한 type의 MyHC이 줄고, 다른 type이 증가하는 패턴을 보이는데, 본 연구에서 MyHC 2b의 증가는 MyHC I 뿐만 아니라 type 2a나 type 2x의 감소에서 기인되었을 가능성도 있을 것이다. 또한, 근육의 재생이나 성장을 담당하는 근육위성세포(muscle satellite cells; Morgan and Partridge, 2003)와 같이 미분화 세포들이 antimycin A에 의해 활성화 되어 새로운 MyHC type 2b myotube들이 형성되었을 가능성도 있었을 것이라 사료된다. 향후 근육 줄기세포나 위성세포 특이 항체를 사용하여 이들 population 변화를 분석할 필요도 있을 것으로 사료된다.
Fig. 2.
(a) Representative Western blot images of C2C12 myotubes treated with different concentration of antimycin A (0, 100, 200, 500, 700, 1,000 ng/mL). Changes in protein expression of myosin heavy chain (MyHC) 2b (b) and MyHC slow (c) in C2C12 myotubes treated with antimycin A (0, 100, 200, 500, 700, 1000 ng/mL). Density of bands from Western blot was normalized by density of Anti-MyHC all. Data are means ± SE of triplicates per group. * p < 0.05 versus control.
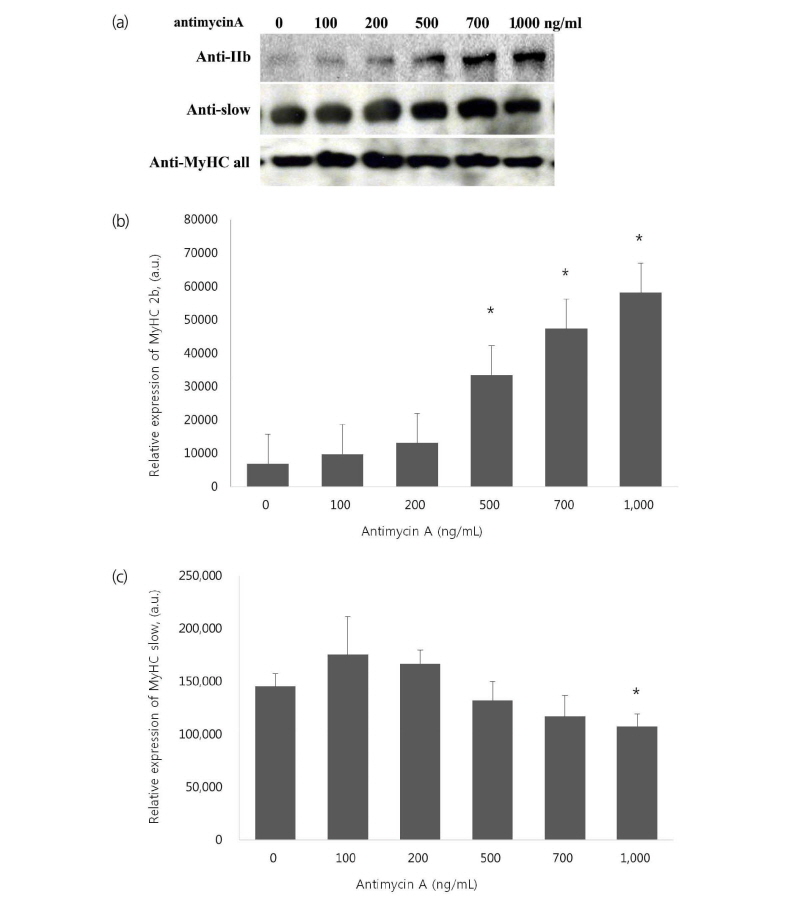
Enzyme activity in energy metabolism pathway
골격근의 형질은 구조단백질의 구성이나 에너지대사의 패턴에 따라 매우 다양하게 나타나는데, MyHC 2x와 2b가 많이 발현되는 빠르게 수축하는 근육에는 phospho-creatine을 이용하여 ATP를 생산하는 creatine kinase와 pyruvate를 이용하여 에너지를 생산하는 lactate dehydrogenase (LDH)의 활성이 매우 중요한 역할을 하는 것으로 알려져 있다(Westerblad et al., 2010). 이에 따라, 근육세포 내 MyHC 2b 단백질 발현 변화와 더불어, antimycin A 처리에 의한 이들 에너지 대사 관련 효소의 활성을 분석하기 위해 C2C12 세포에 antimycin A를 농도 별로 처리한 후 creatine kinase와 LDH 효소활성을 측정하였다. 많은 연구들이 심장근육에서 creatine kinase가 세포 내 에너지 대사에 많은 역할을 하고, 특히 glycolysis와 관련이 되어 있으며, ATP 생성과 더불어 이온균형에 영향을 주는 것으로 알려져 있다(Booth and Clark, 1978, Tepp et al., 2011, Wallimann et al., 2011). 또한, 에너지를 많이 소비하는 조직에서 에너지 균형을 유지 해주고, ATP 생산 및 소비 간 균형에 큰 역할을 한다(Meyer et al., 2006). 같은 연구에서 antimycin A를 이용하여 mitochondria내 electron transport chain의 활성을 저해한 결과 mitochondria내 apoptosis, ischemia reperfusion injury, hyperglycemia induced toxicity등과 관련이 있는 것으로 알려진 reactive oxygen species의 형성이 증가되었지만, creatine kinase활성으로 인한 steady-state ADP recycling을 통해 hyperpolarization은 일어나지 않은 것으로 나타났다(Meyer et al., 2006). 본 연구에서 Antimycin A 처리는 근육세포 내 creatine kinase의 활성에 유의적 영향이 없는 것으로 나타났다(Fig. 3). 근육 내 mitochondria 활성 중에서 hypoxia를 유도하여 부분적으로site 3의 활성을 저해했을 경우는 lactate 생성이 증가하는 것으로 나타났지만, 산소공급을 차단하여 완전히 차단했을 경우에는 glycolysis활성도 일어나지 않은 것으로 밝혀졌다(Dickman and Mandel, 1990). 같은 연구에서, Rotenone을 이용하여 mitochondria site 1을 저해 했을 경우에도 lactate 생성이 증가하였고, antimycin A를 이용하여 site 2를 저해했을 경우 glycolysis의 활성증가를 보이지 않았다. 이러한 산화적 대사(oxidative metabolism)와 glycolysis 활성도의 관계를 보여주는 결과에 비추어 볼 때, respiratory inhibition 상태에서도 세포의 생존을 위해 ATP가 anaerobic glycolysis를 통해 생성이 되는 것으로 해석될 수 있다. 이러한 관계를 확인하기 위해서 antimycin A를 처리한 근육세포 내 LDH의 활성을 측정한 결과, 높은 농도의 antimycin A (500, 700 ng/mL) 처리로 인해 LDH의 활성이 유의적으로 증가되는 것으로 나타났다(p < 0.05; Fig. 4).
Fig. 3.
Changes in creatine kinase activity in C2C12 myotubes treated with antimycin A (0, 100, 200, 500, 700, 1,000 ng/mL). Activity of creatine kinase (CK) was measured by spectrophotometer and indicated as percentage over calibrator where CK activity made 1 µmole phosphate bound to ADP and converted into ATP per minute.
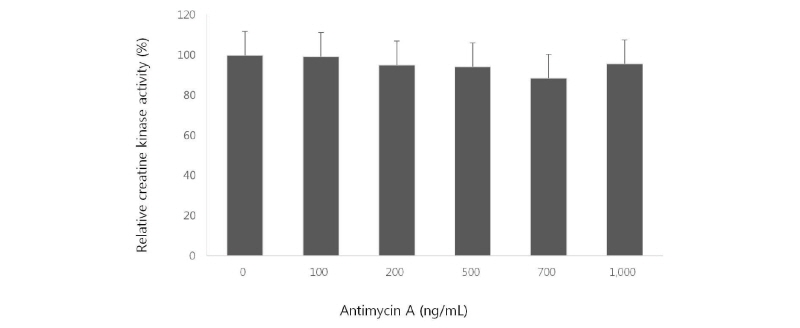
Fig. 4.
Changes in lactate dehydrogenase (LDH) activity C2C12 myotubes treated with antimycin A (0, 100, 200, 500, 700, 1,000 ng/mL). Activity was calculated by measuring oxidation of NAD+ in 1.5 mM pyruvic acid and 0.14 mM NADH, 0.1 M potassium phosphate buffer at 340 nm, 25℃. *p < 0.05 versus control.
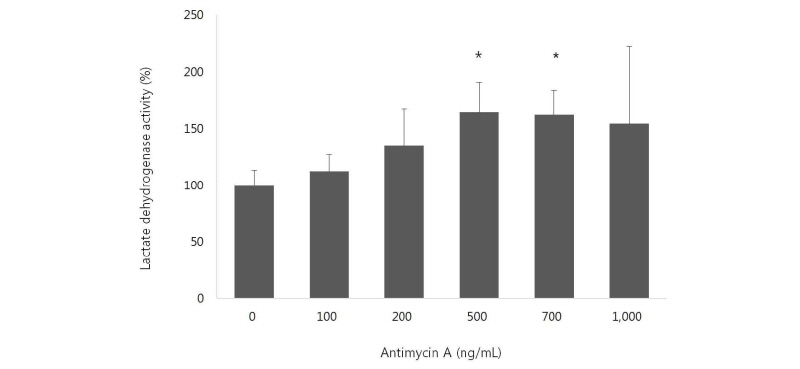
Conclusion
본 연구는 mitochondria의 산화적 인산화 과정을 저해하는 antimycin A를 이용하여 근육세포의 에너지대사 조절을 통해 근육의 형태가 전환되는지를 알아보기 위하여 수행되었다. 근육세포생존에 영향을 미치지 않는 낮은 농도의 antimycin A를 처리했을 때, mitochondria 활성 저해와 더불어 LDH의 활성이 증가함으로써 ATP가 생성되고, 72시간동안의 지속적인 대사 변화가 결국 근육세포 내 MyHC isoform의 발현(MyHC slow → MyHC 2b)에도 영향을 끼칠 수 있다는 것을 알 수 있었다. 이번 연구의 결과는 향후 근육형질과 근육 내 에너지 대사의 상관관계에 대한 메커니즘 연구나 근육 내 다양한 대사관련 인자의 활성 조절을 통한 근육형질변화 유도에 관련된 기초 자료로 쓰일 수 있을 것이라 사료된다.