Introduction
A toxigenic fungal species such as Aspergillus, Penicillium, Alternaria, and Fusarium produce several mycotoxins as secondary metabolites (Abdel Hameed et al., 2012). Generally, the mycotoxins in the contaminated food and feedstuff are produced mainly by those of three fungal genera. Aflatoxins is produced by Aspergillus species, while ochratoxin A is produced by both of Aspergillus and Penicillium species, and zearalenone (ZEN) and fumonisin are produced mainly by Fusarium species (Streit et al., 2012).
Particularly, ZEN is a common mycotoxin that produced by Fusarium species. ZEN is known as a potential estrogenic metabolite contaminating in the food and feed ingredients (Takemura et al., 2007). Recently, the interest of ZEN exposure is increasing to mycotoxin mixtures because of their natural co-occurrence, although it may not always be predicted (Pietsch et al., 2013). ZEN contaminant in livestock animals are influence reproduction system (e.g., ovarian, placenta, and testicular hormone) and infectious diseases via dysregulation of the humoral immune system (Antonissen et al., 2014). The toxicity of ZEN as sensitive mycotoxin is associated with reproduction disorders and hyperestrogenism in pigs, which is the most susceptible species (Kallela and Ettala, 1984). ZEN can cause reproductive disorders of swine because estrogenic and anabolic steroids bind to the estrogen receptors (Vejdovszky et al., 2017). ZEN primarily metabolizes in the liver and its concentrations in the urine indicates the toxic level in the body (Pajewska et al., 2018). ZEN can be easily destroyed by heat or other physical or chemical treatments. For example, fermentation with bacteria and yeast was decreased in ZEN levels (Ryu et al., 2002). ZEN is a not degradationat at 150°C for 44 h. Whereas, ZEN is reduced in contaminated corn after treatment with 3% NH3 at 50°C for 16 h. Ammoniation, propionic acid, and acetic acid had no effect on ZEN levels in yellow corn (Bennett et al., 1980). However, ZEN is destroyed by ammonium persulfate, formaldehyde, 3% ammonium hydroxide, and ozone (Matsuura et al. 1979; Bennett et al., 1980). Similar to deoxynivalenol (DON), ZEN in food and feed causes health problems to humans and livestock animals, thereby leading to economic losses.
Metabolomics has been used for screening the biomarker metabolites in biological systems by nuclear magnetic resonance (NMR), liquid chromatography-mass spectrometry (LC-MS), and gas chromatography-mass spectrometry (GC-MS) (Aretz and Meierhofer, 2016; Nam et al., 2018). Particularly, proton nuclear magnetic resonance (1H-NMR) spectroscopy is a non-destructive method of metabolite profiling as compared to others analytical platforms (Savorani et al., 2013). Moreover, metabolic NMR can identify and quantify the metabolites without refer to standard compounds. In the present study, the NMR analysis aims to assess potential differences of metabolomic patterns in the piglets fed ZEN-contaminated diet.
Materials and Methods
Experimental design of ZEN-treated piglets
The study design was approved by the National Institute of Animal Science Institutional Animal Use and Care Committee (No. 2015-147). Six-week-old male piglets (approximately 19 kg) were purchased from the commercial market. They were housed in separate rooms for acclimation at 25 ± 1°C for one week. The piglets were assigned into control (four piglets per group) and ZEN mycotoxin groups (five piglets per group). A basal diet formulated to meet the nutritional requirements for piglet was fed to the animal in the control group. The ZEN-treated group was fed a mixture of basal diet with ZEN (Biomin Pte.Ltd., Singapore) at 0.8 mg/kg of basal diet. Throughout the experimental period, feed and water were provided ad libitum. ZEN toxicity effect by fed diet was analyzed using ultra performance liquid chromatography (UPLC), as described in a previously study (Reddy et al., 2018).
Sample preparation and NMR analysis
After the experiments, the control and ZEN-treated piglets were slaughtered. Anesthetics used included a barbiturates and pentobarbital. Liver, serum, and urine samples were collected immediately and frozen with liquid nitrogen for metabolomic analysis. For NMR spectroscopy, 45 µL serum or urine sample was mixed in 5 µL deuterium oxide (D2O) containing 20 mM of trimethylsilylpropanoic acid (TSP). Liver tissue samples were pulverized to fine particles using mortar, and then transferred into nanotubes. A 600 MHz Agilent NMR spectrometer (Agilent Technologies, Palo Alto, CA, USA) with high-resolution magic angle spinning (HRMAS) was used for metabolomic analysis in three different tissues. The peaks were conducted by Carr-Purcell-Meiboom-Gill (CPMG) pulse sequence to remove the water and macromolecule signals. The NMR spectra were measured in < 1.704 s acquisition time and 10 min 20 s of total acquisition time. Each peak was identified and quantified with Chenomx NMR Suite 7.1 (Chenomx Inc., Edmonton, AB, Canada).
Statistical analyses
The peaks were aligned with the icoshift algorithm of MATLAB R2013b (The Mathworks, Natick, MA, USA) and multivariate statistical analyses. Principal component analysis (PCA), orthogonal partial least squares discriminant analysis (OPLS-DA), and variable importance in the projections (VIPs) were imported into SIMCA-P+ software (Umetrics, Umea, Sweden). To predict meaningful metabolic pathway, enrichment analyses selected metabolites from the VIPs were performed using MetaboAnalyst 4.0 (http://www.metaboanalyst.ca).
Results and Discussion
Multivariate analysis of liver, serum, and urine metabolites
NMR-based metabolomic analysis was performed in the liver, serum, and urine samples from control and ZEN-treated piglets. The combined PCA score plot within the 95% hotelling T2 ellipse were separated in different samples after 4 weeks of ZEN treatment (Fig. 1A). The characteristics of the PCA model were excellent: R2X = 0.852 and Q2 = 0.537. Using one-dimensional (1-D) NMR spectra, 28, 29, and 20 metabolites were profiled in liver, serum, and urine, respectively. The serum showed a more significant distinction as compared with liver and urine in the PCA score plot. For integrated analysis, glucose, lactate, taurine, glycine, alanine, glutamate, glutamine, and creatine from OPLS-DA were potential compounds for predicted discriminating analysis (VIPs > 1) (Fig. 1B). Heat map by PCA analysis showed changes in potential metabolite compounds (Fig. 2). Phenylalanine and tyrosine showed high concentrations in serum from the ZEN-treated group of piglets, whereas valine showed low concentrations. Formate was increased in urine, while alanine, arginine, choline, and glucose concentrations were increased in liver. Liver is a major tissue for mycotoxin metabolism, which is associated with glycogenolysis and detoxification (Ji et al., 2017). Pig urine had correlation with mycotoxin exposure (Thieu and Pettersson, 2009). Swine urine is potentially the most useful metabolic diagnostic matrix for investigating the relationship between metabolites and a specific pathophysiological status (Merrifield et al., 2011). The mycotoxin showed antagonistic effects on liver and serum metabolites in mice (Ji et al., 2017). In the present study, the potential differences in the metabolic response described the effects of single mycotoxin-contaminated in feed. ZEN toxicity changes the levels of progesterone, red blood cell, and platelets in serum (Chen et al., 2015). The common effects of ZEN were associated with reproductive disorders and hyperestrogenism in pigs (Agag, 2004). The mycotoxins and secondary metabolites were profiled in urine (Ahn et al., 2017). The toxicity levels of zearalenone were measured in feed by high performance liquid chromatography (HPLC) (Kim et al., 2011). Eleven kinds of mycotoxin from the cereals produced in Korea were measured using simultaneous analysis (Ok et al., 2007). The highest concentrations level between DON and ZEN were found in dried corn samples by LC-MS, and climate change caused increase in mycotoxin levels (Kim and Chun, 2009). Rice straw contaminated with mycotoxin was not dependent on the regional area or the storage types (Kim et al., 2013).
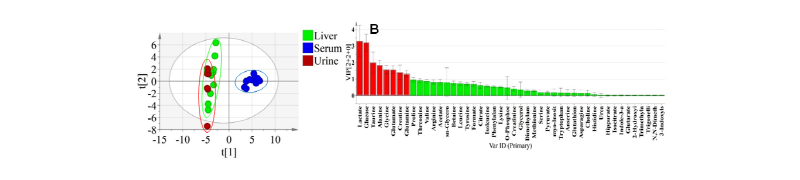
Fig. 1. Principal component analysis (PCA). (A) Score plot: R2X = 0.852 and Q2 = 0.537. (B) The variable importance plots from orthogonal partial least squares discriminant analysis (OPLS-DA) showed that significant metabolites contributed to separation of the clusters in piglets. Six-week old piglets were treated with 0.8 mg/kg Zearalenone (ZEN) for 28 days.
Enrichment pathway analysis of metabolomics
Metabolomic compounds were used in enrichment analysis for identifying the metabolite changes through the website server called MetaboAnalyst. The metabolite set enrichment analysis (MSEA) approach is an effective analytical tool for visualizing integrated metabolomes. Forty-nine metabolites, selected via VIPs, were estimated the mechanism patterns (Table 1). In particular, four pathways were considered the potential mechanism, such as glycine and serine metabolism, ammonia recycling, urea cycle, and glucose and alanine cycle, about ZEN-treated samples (p < 0.05, FDR < 0.1). Glycine and serine metabolism consists of betaine, creatine, dimethylglycine, glycine, glutamic acid, alanine, threonine, serine, pyruvic acid, and methionine. Ammonia cycle consists of glycine, glutamic acid, asparagine, histidine, serine, pyruvic acid, and glutamine compounds. Urea cycle consists of glutamic acid, alanine, urea, arginine, glutamine, and pyruvic acid. Glutamic acid and glycine were associated with the ZEN toxicity. Finally, it could be suggested that the predicting metabolisms can provided the correlation among biomarkers by ZEN treatment.
Conclusion
NMR-based metabolome profiling provided insights into the effects of mycotoxin metabolism-related compounds for ZEN-treated piglets. NMR was used for studying differential metabolomic patterns and screening metabolites from different tissues of piglets fed with ZEN-contaminated feed. The metabolite compounds of liver in ZEN treatments were similar to serum. However, metabolites in serum were clearly differed. The integrated metabolic pathways suggested that ZEN-contaminated diet provided the different metabolomic patterns in piglets. Therefore, potential biomarker compounds and pathways can be considered for a better understanding of how the ZEN-contaminated feed affect the liver, serum, and urine in piglets.
Acknowledgments
This work was carried out with the support of “Cooperative Research Program for Agriculture Science and Technology Development (Project No. PJ01093202)” and the 2018 RDA Fellowship Program of the National Institute of Animal Sciences, Rural Development Administration, Republic of Korea.