Introduction
Plant-pathogen interactions are a subject of great complexity exhibited by both organisms. Pathogens have evolved a variety of ways to infect its host. A pathogen can only cause a successful infection by suppressing host immunity (). On the other hand, plants over time have evolved numerous strategies (immunity) to encounter the pathogen’s attack. Plant immunity can be broadly classified into two distinct categories (; ). The first barrier in the line of defense is the cuticle and waxy layer that prevent the entry of a pathogen. Next, is a highly complex and sophisticated defense mechanism in plants known as the innate immune system (). The most salient feature of the plant immunity system is PAMP (a plant initially senses microbes via perception of pathogen associated molecular patterns) which is responsible for the recognition of the structural components of pathogens such as flagellin and other similar structures (; ). This first level of innate immunity leads to PTI (PAMP triggered immunity), which primarily has a role in preventing infection (). Upon recognition of a pathogen, PTI is responsible for secreting ion fluxes across the plasma membrane, mitogen-activated protein (MAP) kinase activation, production of reactive-oxygen species, rapid changes in gene expression and cell wall reinforcement to combat the pathogen’s attack (; ; ; ). Several pathogens are capable of evading PAMP triggered immunity by suppressing all the PAMP recognition receptors by secreting virulent effectors (). However, through the process of parallel co-evolution between plants and pathogens, some plants have evolved resistance proteins (R proteins) to identify these effectors, also known as Avirulent (Avr) effector proteins (). Some effectors, called Avr proteins, have specific gene-for-gene interactions with host resistance proteins (). This process of recognizing an Avr effector is also called effector-triggered immunity (ETI) which primarily triggers local cell death known as the hypersensitive response (HR). Subsequently, pathogens have also evolved effectors capable of suppressing ETI, and so, the battle between hosts and pathogens goes on (; ; ; ).
The oomycetes are hemibiotrophs and have been accepted as forming a class of heterotrophic organisms, probably derived from heterokont algal ancestry and usually are discussed with other heterotrophs within the fungi (69; ). They show, in general, a similar level of organization to both Chytridiomycetes and Zygomycetes but otherwise, appear unrelated to either of these classes (). Oomycetes are known as one of the most destructive pathogens among the plant kingdom. Over the years, oomycetes have been found to be the prime cause of various epidemics, such as the Irish potato famine of 1845, as well as causing economic losses to crops all over the world (; ; ). Oomycetes secrete many proteins with prospective effector activities (; ; ; ; ). Four oomycete Avr genes have been cloned, two from the Phytophthora species and two from Hyaloperonospora parasitica (; ). The encoded proteins share little sequence similarity except for two conserved motifs RXLR and dEER at the N terminus (). The exact function of these motifs is still unclear. This review is an attempt to summarize the structural and functional findings with respect to the RXLR domain of Avr effectors from oomycetes. The underlying assumption of this review is that the RXLR domain is not involved in effector activity but instead facilitates secretion or entry of the effector into its target. Future studies will unravel more information related to this domain.
Effectors of oomycetes
Oomycetes secrete effectors through the general secretory pathway and infection structures, such as haustoria (). Oomycete effectors retain an N-terminal signal peptide which can function extra- or intracellularly. Oomycetes secrete apoplastic effectors in the extracellular vicinity of plant cells. Hundreds of apoplastic effectors have been reported that are involved in knocking down the entry gateway for pathogens (; ).
Apoplastic effector
The “arms race” has resulted in various strategies for the adaptation, defense and counter defense between pathogens and hosts. Furthermore, this arm race has been driving antagonistic co-evolution and producing the evolutionary changes that are profiling effectors and their host targets (; ). In this whole diversified selection, oomycetes have developed a variety of apoplastic effectors (). According to numerous findings, oomycetes are known to possess the following apoplastic effectors: pathogen related enzyme inhibitors (), elicitins (), Nep1-like proteins (), transglutaminase (), and CBEL (Cellulose Binding, Elicitor and Lectin like proteins) (). Apoplastic effectors are involved in a wide array of functions including the following: inhibiting plant defense related enzymes; binding sterol (elicitins, sterol carrier protein); functioning as toxins, and triggering host tissue necrosis thereby facilitating the infection. (; ; ; ; ).
Cytoplasmic effectors
Crinkler (CRN) gene family
CRN effectors, also known as crinklers, are cytoplasmic in nature and form a complex family of proteins (about 400 - 850 amino acids) (). All CRN effectors possess a conserved N-terminal domain involved in a translocation activity and a complex C-terminal domain with diverse effector activities (). Initially, CRNs were known for causing crinkling and cell death in infected plants (). Now, CRNs have been found to be involved in manipulating profound host cellular processes, similar to other effectors from bacteria causing phenotypical abnormalities as necrosis, chlorosis, and tissue rotting (). Additionally, one CRN gene (crn8) has been reported to possess a RD kinase domain (; ).
RXLR gene family
During the last few decades, a large number of RXLR effectors from oomycetes have been identified that are avirulent in nature. On the other hand, plant R genes that give resistance against a diverse group of oomycetes have also been identified. Avirulence proteins trigger ETI in their host; in contrast, these effectors can also be virulent in nature in susceptible host genotypes. This whole phenomenon follows the gene model, inferring that the plant active defense is activated when a plant resistance (R) gene identifies the intrusion of an Avr effector ().
The largest class of effectors found in oomycete genomes contains Avr genes (Kamoun et al., 2015). These diverse effectors are therefore avirulence gene homologs (Avh) (). They are called RXLR-class effectors after the characteristic N-terminal motif found in most members of this group. Four RXLR effector proteins were originally isolated from oomycete pathogens by map-based cloning: Avr1b-1 from Phytophthora sojae (), Avr3a from P. infestans, and ATR13 and ATR1NdWsB from H. arabidopsidis, the downy mildew of Arabidopsis (; ; ; ). Studies have revealed that these proteins possess an N-terminal signal peptide and an RXLR motif (). This integrated structure with a N-terminal signal peptide and the RXLR motif, also known as the targeting domain, is crucial for translocation of the effector protein into plant host cells, and a C-terminal ‘functional’ or ‘effector’ domain (; ; ). The RXLR motif is analogous to the RXLX motif that is essential for the translocation of proteins from malaria parasites (Plasmodium spp.) to host cells (; ). Corresponding to the other fungal avirulent genes, these effectors are also secreted in the host cytoplasm which is leads to R-gene-related cell death. The recognition of the effectors seems to take place inside plant cells, implying that these pathogen proteins are delivered across the plant membrane during infection (; ). Subsequently, genome sequence interrogation based on the conserved features of these Avr proteins, particularly the presence of an RXLR motif, has led to the identification of further Avr genes: Avr1a and Avr4/6 from P. sojae () and Avr4 (), Avrblb1 () and Avrblb2 (Oh et al., 2009) from P. infestans.
Structure of Avr effectors
During the last two decades, there has been a tremendous amount of research done related to the structural analysis of Avr effectors. As a result of these numerous findings, a model has been proposed that defines the structure and activity of RXLR effectors (). According to this emerging view, the N-terminal domain of Avr effectors contains a short sequence motif just downstream of the signal peptide RXLR. The RXLR motif is characterized by a region of 25 - 30 amino acids that are essential for host-cell translocation and targeting (). The RXLR motif is followed by the EER motif (less than 25 residues) that resides downstream of the RXLR motif. Additional amino acids flanking the conserved core have also been reported (; ). Numerous studies have indicated the sequence and position similarity of the oomycete Avr effectors’ conserved RXLR motif with the RXLX E/D/Q motif, involved in the translocation of proteins from the malaria parasite Plasmodium falciparum into the host erythrocytes (; ; ) (Fig. 1).
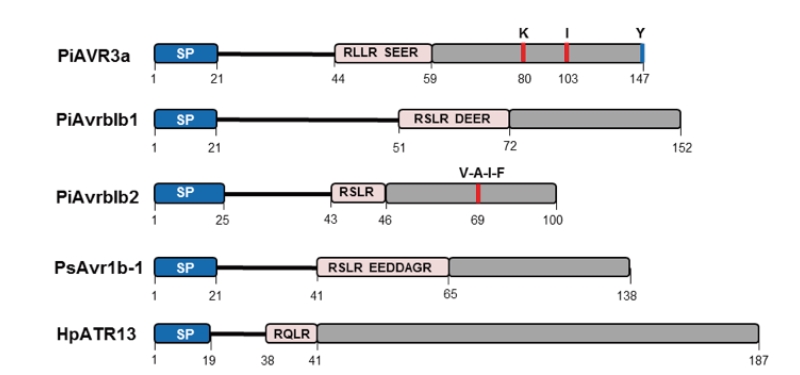
Fig. 1. Domain organization of cytoplasmic RXLR effectors. Schematic drawings of PiAVR3a of Phytophthora infestans (Bos et al., 2006), PiAvrblb1 and PiAvrblb2 of P. infestans (Oh et al., 2009; 2010; Vleeshouwers et al., 2008), Avr1b-1 of P. sojae (Shan et al., 2004), and HpATR13 of Hyaloperonsopora parasitica (Allen et al., 2004). The numbers under the sequences indicate the amino-acid positions. The highlighted RXLR domain includes the RXLR sequence itself and the downstream dEER sequence. The gray colors distinguish the regions of the effector proteins that are involved in secretion and targeting from those involved in effector activity (Modified from Morgan and Kamoun, 2007 and Oh et al., 2010).
In contrast, the variation in the consensuses model of the N-terminal domain of Avr effectors has also been reported. Variations in the RXLR domain have been reported for H. arabidopsidis ATR5 with the absence of the canonical RXLR motif (RVRN instead of RVLR in related effectors) and cucurbit downy mildew, Pseudoperonospora cubensis ATR1 and AVR3a, with an R to Q substitution in the first residue of the motif QXLR (). Consequently, these reports have proposed that these variations of the RXLR motif potency are common in circulating oomycetes; however, further study on the subject is required.
Recently, bioinformatics have also had a pivotal role in unreeling the structural nature of Avr effectors. However, in silico/3D structural analysis is specifically effective in analyzing the C-terminal region of the effectors (; ). Recent studies have revealed five 3D structures including AVR3a4 and AVR3a11 from P. capsici (), PexRD2 from P. infestans (), and ATR1 and ATR13 from H. arabidopsidis (). These studies have shown the RXLR domain as a disoriented structure, suggesting that the N-terminal domain RXLR-EER motif possibly attains a disorderly conformation, which can be a significant molecular feature in host cell translocation ().
The C-terminal domains of RXLR effectors have been reported to carry conserved sequence motifs termed ‘(W)’, ‘(Y)’, and ‘(L)’ (). Moreover, the structures of the AVR3a homologues, PexRD2 and ATR1, show that the C-terminal domain of RXLR effectors share a common alpha-helical protein fold (), termed the WY domain (; ), a complex of the earlier reported W and Y motifs (; ). Boutemy et al. (2011) have proposed the 3D structural nature of the C-terminal domain of RXLR effectors from P. capsici AVR3a11, a homologue of the P. infestans AVR3a and Avr1b from P. sojae (). According to the proposed model, the two effectors do not show a substantial sequence similarity. However, they have prolific similarity in α -helical fold in their structure (). The research findings not only propose a model for the core α -helical fold (termed the ‘WY-domain’) that provides molecular stability to the overall structure of the protein but also propose that the WY-domain is responsible for plastic evolution which promotes effector virulence in pathogens ().
Role of the RXLR domain
The role of RXLR domain had been a subject of controversies and differences. Until now, various models for the translocation of RXLR effectors have been presented; their exact mechanism is still unclear and debatable (). According to most widely accepted models related to the function of the RXLR domain, the RXLR and DEER motifs have been accepted as being involved in the translocation and entry of effectors into plant cells (; ; ; ; ; ).
Several experimental findings have provided information suggesting the RXLR domain to be prerequisite for effective effector transfer during infection in the case of P. sojae and P. infestans (). The first report interrogating the entry mechanism of Avr1b into plant host cells was established by mutagenesis trials published by Dou et al. (2008). Introducing mutations in the RXLR-DEER motifs resulted in a poor translocation activity and not entry into the host cells, leaving the mutant RXLR effector in the apoplastic space in the case of the P. sojae effector Avr1b (). The results were further validated by (Kale and Tyler, 2011) in the case of the effector (Avr3a) of P. infestans. Whisson et al. (2007) performed similar mutagenesis experiments based on a loss of function infection assay. The research findings revealed that the RXLR–DEER motifs are involved in the translocation and entry of AVR3a into the host cells (). In addition, experiments have also been performed to interrogate pathogen independent effector entry inside the host cell. The research findings have reported that the RXLR effectors of oomycetes and fungi are capable of translocating into a host cell in the absence of the pathogen (; ).
Confirmation studies have also been conducted to validate if the RXLR motif has any role in triggering the host immune response. In the case of P. infestans, structure-function analysis of the Avrblb2 family has indicated that a 34–amino acid region in the C-terminal of Avrblb2 is sufficient for triggering Rpi-blb2 hypersensitivity and that a single positively selected Avrblb2 residue is critical for recognition by Rpi-blb2, thus suggesting no role of the RXLR domain in triggering the host immune response (Oh et al., 2010)(Fig. 2). Furthermore, the RXLR domain in association with endocytosis and phospholipid binding translocation models have been rejected because lipid binding of the effector has been found to be mediated by the C-terminal positively charged lysine rather than the N-terminal lysine (; ; ).
Recently, a new striking report published by Wawra et al. (2017) did an RXLR domain function analysis. The findings of this report have started debate on the function of the RXLR motif, making the function of the RXLR domain once again yet more controversial. According to the research findings, the RXLR motif is involved in the secretion of the effector (AVR3a) from haustoria rather than in the translocation of the effector (). The striking evidence provided by the research supporting the RXLR domain secretion model states that AVR3a is intracellularly cleaved and N-acetylated followed by its secretion from haustorium. Thus, these results suggest that the removal of the N-terminal RXLR motif through cleavage is prerequisite for releasing the effector from the pathogen (). The complete cleavage of the RXLR motif prior to entry into the host cell is further validated by previous research findings on C-terminal phospholipid binding rather than N-terminal binding. (; ; ).
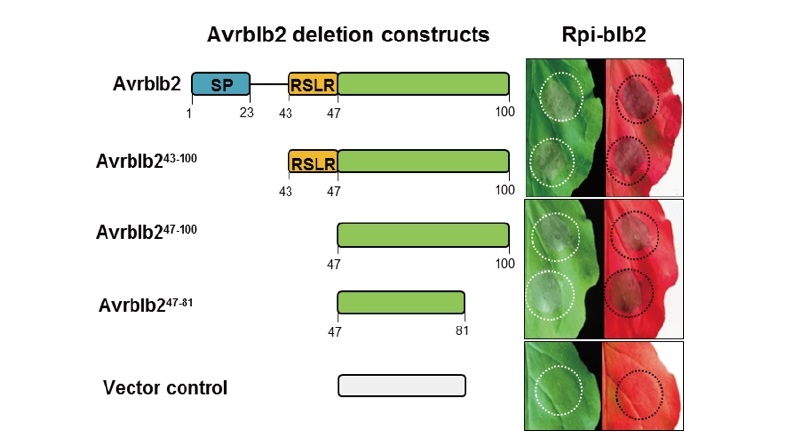
Fig. 2. The 34-amino acid C-terminal region of AVRblb2 (Avrblb247-81) is sufficient for activation of Rpi-blb2–mediated hypersensitive cell death (HCD). Various AVRblb2-deletion constructs were co‐expressed with Rpi-blb2 by agro‐infiltration in Nicotiana benthamiana to determine the AVRblb2 domains required for the induction of the Rpi-blb2‐mediated HCD. A schematic view of the various deletion constructs is shown on the left. Symptoms of infiltration sites co‐expressing the AVRblb2 constructs with Rpi-blb2 are shown on the right (Modified from Oh et al., 2009). Photographs of symptoms were taken 5 days post-infiltration. SP, signal peptide.
Perspectives
Recently, tremendous advances have been made in the field of the effector secretome of oomycetes. The genomic database has been helpful in elucidating the biology of the RXLR effectors. However, numerous challenges still prevail in understanding the functional mechanism of RXLR effectors. In contrast to the previous belief that the RXLR domain is involved in translocation, new research assigning a secretion role to this domain has presented a new challenge in understanding the functional mechanism of effectors. Thus, there is urgent need to revise the functional model of the RXLR domains because discovering the molecular function of the RXLR domain is crucial for a mechanistic understanding of pathogens.