Introduction
Honey is a natural sweet substance which is produced by honey bee from various origins, e.g. nectars of blossoms, secretion of living plants, and excretions of plant sucking insects, and thus shows diverse physicochemical parameters, e.g. sugars, ash, free acidity, colors, polyphenols, vitamins, and amino acids. The international standard by Codex Alimentarius (2001) and the council directive by EU (2002) were established to specify honey type and composition criteria for honey, which can be sold under given names and the rules on labeling, presentation, and information on origins. The food code legislated by Ministry of Food and Drug Safety of Korea (MFDSK, 2019) includes definition, classification, and physicochemical parameters of honey products. The standards and regulations were established to prevent adulteration and mislabeling of honey and to protect its safety and quality. However, there are discrepancies between the standards and regulations in the type of honey and its physicochemical parameters due to different beekeeping conditions among the countries. Legislations cannot always follow the complexity of honey variations, so there are cases where the parameters of authentic and unprocessed honey fail to comply with the composition criteria of regulations, so beekeepers are subject to financial loss in distribution of their products (Thrasyvoulou et al., 2018).
Codex Alimentarius (2001) and EU (2002) classified the types of honeys according to origin into blossom honey from nectars of plants and into honeydew honey from excretions of plant sucking insects (Hemiptera) on the living part of plants or from secretions of living parts of plants. Honeydew honey gets attention from consumers and beekeepers, because of its potential therapeutic potential and claims high market price in Europe and Asia. However, distribution of honeydew honey in the Korean market may be hindered, because the fructose and glucose content (F + G) of the honeydew honey has been known to be lower than blossom honey. The food code established by MFDSK (2019) specifies that the F + G of honey should not be less than 60% regardless botanic origin. However, Codex Alimentarius (2001) and EU (2002) required that the F + G of blossom honey and honeydew honey should not be less than 60 and 45%, respectively. Blossom honeys such as chestnut, acacia, and multifloral honeys are currently distributed and sold at the commercial markets in Korea. Production of honeydew honey, which has been negligent before, is recently increasing because of longer period of hot dry weather during summer.
Multivariate analysis of physicochemical parameters has been used widely as alternative criteria to pollen analysis for classification of honeydew and blossom honeys. Honeydew and chestnut honeys are characterized with dark color, high values of electrical conductivity, pH, and acidity, and high contents of ash, raffinose, melezitose, phenolic, and flavonoid. Acacia honey is light colored honey with the physicochemical parameters opposite to the honeydew and chestnut honeys (Devillers et al., 2004; Pichichero et al., 2009; Kropf et al., 2010; Pita-Calvo and Vázquez, 2017). Mutifloral honeys show diverse colors from yellow to brown and various physicochemical parameters depending on the plant species (Bertoncelj et al., 2011).
The objectives of this study were to characterize physicochemical parameters of the honeydew, chestnut, multifloral, and acacia honeys and to investigate whether the parameters corresponded with the composition criteria for honeys in the related regulations and whether the principal component analysis of the parameters differentiated the four types of honeys.
Materials and Methods
Honey sample
The honey samples used in this study were 10 honeydew, 10 chestnut, 10 multifloral, and 5 acacia honeys which were produced throughout the regions of South Korea during the years from 2016 to 2017. The samples were the reference honeys purchased from the beekeepers and stored by Korea Beekeeping Agricultural Cooperative. Their botanic origins were specified by the beekeepers regarding floral sources and were confirmed by means of sensory analysis including aroma, taste, and, color and carbohydrate composition analysis at the research laboratory of the cooperative. The honey samples were stored at 4℃. The sugar crystals, if found in the honey, were solubilized at 40℃.
Moisture content
The moisture content of honey was determined by using the refractometer for honey moisture (Master-honey, Atago, Japan).
Free acidity
Ten grams of honey sample were dissolved in 75 mL of distilled water and titrated with 0.1 N sodium hydroxide using phenolphthalein as acid base indicator. The free acidity was calculated using the equation 1 as follows.
Free acidity (meq kg-1) = 0.1 N sodium hydroxide (mL) × factor × 100/10 g (1)
Carbohydrate composition
Approximately one gram of honey was accurately weighed, dissolved in 25 mL of distilled water, and put into 100 mL volumetric flask. Distilled water was added to the graduation mark. The diluted honey solution was analyzed using HPLC to determine contents of fructose, glucose, and sucrose. The HPLC system (1200 series, Agilent, Santa Clara, USA) which was equipped with the carbohydrate column (Waters, Milford, USA) was used to deliver the elution solvent of acetonitrile and water (75 : 25) with the flow rate of 1 mL/min. The sugars in the elution solvent were detected using the refractive index detector.
Ash content
Approximately 3 g of honey were accurately put into porcelain crucible vessel and carbonized at 145℃ for 3 h and at 165℃ for 9 h and then ashed at 550℃ for 15 h. The weight of ash was divided with the weight of the honey to calculate the percentage of ash.
Color analysis
The colors of the honeys were quantified with CIE L* a* b*, which was defined by the Commission Internationale de l'Eclairage (CIE, International Commission on Illumination) in Robertson (1976). It expresses color as three values: L* for the lightness from black (0) to white (100), a* from green (-) to red (+), and b* from blue (-) to yellow (+). The CIE L* a* b* and absorbance of honey were determined following the procedure described by Bertoncelj et al. (2007). Forty-five gram of honey was placed on a disposable plastic petri dish with the diameter of 9 cm. The petri dish with the plastic cover was placed on a white paper. The CIE L* a* b* were determined by using the colorimeter (CR-200B, Minolta, Tokyo, Japan). The net absorbance of honey, the difference between absorbances at 450 nm and 270 nm was measured to determine color spectrophotometrically following the procedure of Bertoncelj et al. (2007). 50% (w/v) honey solution was centrifuged at 10,000 × g for 15 min and filtered to remove any coarseㄴ particle and the supernatant was used to measure absorbance at the wavelength of 450 nm and 270 nm. The net absorbance was calculated by subtracting absorbance at 450 nm from absorbance at 720 nm. The results were expressed as mAU.
Statistical analysis
The physicochemical parameters of honeys were determined in duplicate. The mean values of the duplicate measurements were used for statistical analysis. The physicochemical parameters were compared between honeydew, chestnut, multifloral, and acacia honeys by performing one-way analysis of variance followed by Duncan’s multiple range test. The Pearson’s correlation coefficients between the parameters were calculated by performing Pearson product moment coefficient analysis. Honeydew, chestnut, multifloral, and acacia honeys were differentiated by performing principal component analysis of the physicochemical parameters of the honeys. The above statistical analyses were performed by using Sigmaplot (version 13, Systat Software, Chicago, USA).
Results and Discussion
The honeys examined in this study were 10 honeydew, 10 chestnut, 10 multifloral, and 5 acacia honeys which were selected as reference honeys based on beekeeper’s claim, organoleptic testing, and carbohydrate analysis by the research laboratory of the Korea Beekeeping Agricultural Cooperative. The fructose and glucose content (F + G) and fructose to glucose ratio (F/G) were used as key parameters to determine the botanic origins.
Composition criteria for honey
The composition criteria for honeys were established by the related agencies (Codex Alimentarius, 2001; EU, 2002; MFDSK, 2019) to facilitate distribution of the honey products without misleading consumers. The hydroxymethylfurfural (HMF) contents, and stable carbon isotope ratios of all the honeys in this study were less than 5 mg/kg and - 24 per mille, respectively (results not shown), which indicated that the honeys were fresh with minimal heat treatment and not adulterated with cane sugar. Codex Alimentarius (2001) and EU (2002) limited HMF content to 40 mg/kg, whereas MFDSK (2019) limited it to 80 mg/kg. The stable carbon isotope ratio was determined to detect adulteration of honey with cane sugar. The food code from MFDSK (2019) specifies that the stable carbon isotope ratio of honey shall not be more than - 22.5 per mille, but there is no limit set by Codex Alimentarius (2001) and EU (2002).
Table 1 showed that the values of moisture contents and sucrose contents of all the honeys were not more than 20 and 7%, respectively, which indicated that all the honeys were properly concentrated and aged to maintain shelf stability and acceptable quality. The composition criteria of moisture content and sucrose content for the honeys specified in the council directive (EU, 2002) are not more than 20 and 5 - 10%, respectively, whereas those in the food code (MFDSK, 2019) are not more than 20 and 7%, respectively. The moisture content of more than 20% may cause microbial growth during distribution and storage of honey. The sucrose content more than 7% may indicate a premature honey in which sucrose from the nectar is not converted properly into fructose and glucose during aging or reveal possible adulteration with commercial sugar syrup during its production.
The free acidity of honey is a parameter that can assist in assessing deterioration level of honey. Table 1 showed that the mean values of free acidities of the honeydew, chestnut, multifloral, and acacia honeys were 31.2, 23.1, 19.8, and 13.5 meq/kg, respectively. The highest value of free acidity of all the honeydew honeys was 39.4 meq/kg, which was close to 40 meq/kg, the maximum limit in the food code (MFDSK, 2019). Krauze and Zalewski (1991) reported that the free acidities of honeydew, multifloral, and acacia honeys from Poland were 33.12, 27.99, and 15.16 meq/kg, respectively. Devillers et al. (2004) reported that the free acidities of fir honeydew, chestnut, and acacia honeys were 24.24, 12.20, and 8.954 meq/kg, respectively. Bertoncelj et al. (2011) reported that the free acidities of honeydew honeys from fir and spruce, forest honeydew honey, chestnut honey, and acacia honey were 20.1, 30.9, 28.5, 13.3, and 14.4 meq/kg, respectively. These results as well as our results showed that honeydew honeys generally had higher free acidities than blossom honeys. Considering high free acidity of honeydew honey, the maximum limit of free acidity established at 40 meq/kg by MFDSK (2019) shall be changed to 50 meq/kg, which is specified for honeydew honey by Codex Alimentarius (2001) and EU (2002).
Carbohydrate composition
The composition of carbohydrate differs between blossom honey and honeydew honey and is a valuable parameter to differentiate the honeys. Table 1 showed that the mean values of F + G of the acacia, multifloral, chestnut, and honeydew honeys were 70.0, 70.0, 65.9, and 57.1%, respectively. The mean values of F/G, 1.68 and 1.67, of the chestnut and acacia honeys, respectively, were significantly at the probability level less than 0.05 higher than 1.23 of the multifloral honey, which was then higher than 1.00 of the honeydew honey. It was noticeable that the standard deviations of F + G and F/G of the multifloral honey were 2.4% and 0.07, respectively, which were similar with those of the other honeys, even though the multifloral honeys of diverse colors originated from various plants. These results indicated that the honeydew honey produced in Korea was not eligible for domestic sale, because its F + G was lower than 60% which is specified in the food code.
Seraglio et al. (2019) reported in their review that the F + G and F/G of honeydew honeys from various geographical origins and botanical origins ranged from 59.9 to 79.7% and from 1.1 to 2.8, respectively. Rybak-Chmielewska et al. (2013) reported that the F + G and F/G of Abis alba honeydew honeys from Poland ranged 58.2 - 67.4% and 1.1 - 1.5. The Abis alba honeydew honeys originated from aphids, mainly Cinara pectinatae (Nordil.), which occurs in temperatures from 25 to 30℃ under favourable weather condition in July and August. Devillers et al. (2004) reported that the F + G and F/G of the fir honey (n = 57) produced in France was 59.00% and 1.302, respectively, and the F + G was lower than the other monofloral blossom honeys. Krauze and Zalewski (1991) reported that the F + G and F/G of honeydew, multifloral, and acacia honeys from Poland were 64.97, 72.60, and 69.09% and 1.101, 1.080, and 1.552, respectively. Ozkok and Sorkun (2018) reported that the F + G and F/G of oak (Quercus frainetto, Ten.) honeydew honey from Turkey ranged from 40.5 to 48.2% and 1.01 to 1.23, respectively. These reports showed that the F + G and F/G of many honeydew honeys were generally lower than those of blossom honeys.
Several studies (Kim et al., 2014; Jang et al. 2016; Jung and Chon, 2016) showed the carbohydrate compositions of acacia, chestnut, and multifloral honeys produced in Korea, but there has been no report on honeydew honey. Jang et al. (2016) analyzed 15 acacia honeys, 15 multifloral honeys, 10 chestnut honeys from beekeepers and commercial markets in Korea. The F + G and F/G of acacia, multifloral, and chestnut honeys were 71.2, 71.2, and 63.2% and 1.59, 1.45, and 1.90, respectively. Kim et al. (2014) analyzed honeys from Korean commercial markets and reported that the F + G and F/G of 10 acacia, 10 multifloral, and 10 chestnut honeys were 72.79, 67.32, and 65.79% and 1.53, 1.47, and 1.95, respectively. Jung and Chon (2016) analyzed 2,987 acacia, 712 multifloral, and 97 chestnut honeys from Korean beekeepers and reported that the F + G and F/G of acacia, multifloral, and chestnut honeys were 71.0, 67.5, and 65.9% and 1.49, 1.34, and 1.75, respectively. These studies as well as our study showed that the F + G of chestnut honeys were consistently lower than acacia and multifloral honeys and the F/G of chestnut and acacia honey were higher than that of multifloral honeys.
Fig. 1 showed that the honeys in this study could be classified based on F + G and F/G into three discernible clusters of honeydew honeys, multifloral honeys, and a mixture of chestnut and acacia honeys. Since the colors of the acacia and chestnut honeys were distinguished visually, the F + G and F/G only without any other physicochemical parameters seemed to make it possible to distinguish between the four types of honeys. Table 1 and Fig. 1 showed that the F + G and F/G of the honeydew honeys were lower than those of the blossom honeys including the chestnut, multifloral, and acacia honeys.
The Korea Institute of Animal Products Quality Evaluation (KAPE, 2013) runs the honey grading exhibition program for improving honey quality and distribution. One of the composition criteria for grading of honey in the program is F/G. According to the criteria of the program, the F/G of acacia and chestnut honeys and multifloral honey of premium grade should not be less than 1.5 and 1.3, respectively. It seems that the F/G may be an indicator for detecting mixing of the blossom honeys with honeydew honeys.
The F/G may also affect the physical characteristics of honeys (Seraglio et al., 2019). In general, crystallization of honey is slow when F/G is higher than 1.3 and is fast when the ratio is less than 1.0. Thus, the acacia and chestnut honeys in this study shall be resistant to crystallization and may remain liquid during storage, whereas the honeydew honey may become solid quickly due to crystallization.
Ash content
Ash content is considered as a quality criterion for botanic origin of honey. The blossom honeys have lower ash content than honeydew honeys. The ash content of blossom honey is ≤ 0.6%; the value of honeydew or blend of honeydew and blossom honey or chestnut honey is ≤ 1.2%. Since the ash content is related closely with electrical conductivity, it is generally replaced by the measurement of electrical conductivity (Ouchemoukh et al., 2007). The mean values of the ash contents of honeydew, chestnut, multifloral, and acacia honeys in this study were 0.718, 0.736, 0.316, and 0.115%, respectively (Table 1). Krauze and Zalewski (1991) reported that the ash contents of honeydew, multifloral, and acacia honeys from Poland were 0.449, 0.226, and 0.033%, respectively. Kropf et al. (2010) reported that the ash contents of chestnut and acacia honeys from Slovenia were 0.66 - 0.72% and 0.05 - 0.06%, respectively.
Color analysis
Visual comparison, spectrophotometric measurement, and color space, such as CIE L* a* b*, can be useful complementary tools for determining the botanic origin of honey (Bertoncelj et al., 2007) and for assess mixing light honey, e.g. acacia honey, with dark honey and vice versa in the honey grading program by KAPE (2013). The colors of the honeys described by visual observation in this study ranged from pale yellow to dark brown. The honeydew and chestnut honeys had dark brown color with different level of lightness. The multifloral honeys had various colors from pale yellow to dark brown. The acacia honeys had various levels of yellow colors. In this study, the mean value of CIE L* for acacia honey was 75.77 indicating light honey. The honeydew and chestnut honeys were dark honeys with CIE L* values of 55.83 and 57.51, respectively. The mean value of CIE a* of the acacia honey were - 0.34 indicating slight greenness. The mean value of CIE a* of the honeydew and chestnut honey were 8.14 and 9.19, respectively, indicating strong redness. The mean value of CIE b* of the acacia honey was 2.52 indicating weak yellowness and those of the honeydew and chestnut honey were 16.15 and 19.17, respectively, indicating strong yellowness. The standard deviations and ranges of the CIE color parameters of the multifloral honey were higher than those of the other honeys. These results indicated that the color of the acacia honey was light yellow with greenish tinge and those of the honeydew and chestnut honeys were dark brown with reddish tinge. The multifloral honey had various colors ranging from light yellow with green tinge to dark brown with reddish tinge.
Honey color was also determined by spectrophotometric measurement and net absorbance was calculated by subtracting absorbance at 450 nm from absorbance at 720 nm. The colors of lights with wavelength at 720 nm and 450 nm are red and blue, respectively. The net absorbance may indicate level of redness. The mean values of net absorbance of the honeydew, chestnut, multiflora, and acacia honeys were 625, 754, 275, and 51 mAU, respectively, meaning that the colors of the honeydew and chestnut honeys had strong redness and the color of the acacia honeys had slight redness. The high standard deviation and range of the net absorbance for the multifloral honeys indicated various levels of redness.
Pearson’s correlation coefficient
Pearson product moment correlation analysis was performed between the parameters shown in Table 1. Several Pearson’s correlation coefficients in bold letters in Table 2, which were either higher than 0.9 or lower than - 0.9 and also significant at the probability level less than 0.01, were found between the physicochemical parameters, such as ash content, CIE L*, CIE a*, and net absorbance. The ash content of the honey was strongly correlated positively with CIE a* and net absorbance and negatively with CIE L*. The results indicated that the honeys with higher ash contents had darker red colors than those with lower ash contents. These results suggested that either mineral ion itself or complexes of mineral ion and organic compounds might be responsible for the honey colors, particularly redness.
The Pearson’s correlation coefficient between CIE a* and CIE L* which was higher than that between CIE b* and CIE L* suggested that CIE a* might be a better parameter than CIE b* for differentiating the honeys. The results indicated that color variation from redness to weak greenness was related closely with lightness variation from black to light. The high correlation coefficients between the net absorbance and the CIE color parameters indicated that the net absorbance of the honeys might be an alternative parameter to measure honey color. However, CIE Lab system have advantage to measure a color to get three CIE color parameters of L*, a*, and b*, which may make it possible to express more accurate color than a single spectrophotometric absorbance value.
Principal component analysis
Principal component analysis (PCA) was performed to evaluate physicochemical parameters for differentiating the honeydew, chestnut, multifloral, and acacia honeys. Since the moisture contents were not significantly different between the honeys and the net absorbance was an inferior alternative to the CIE color parameters, we disregarded the two parameters of moisture content and net absorbance from the PCA. Table 3 showed the percentages of the total variance which the principal component 1 (PC1) and the principal component 2 (PC2) accounted for, when the various parameters were subjected to PCA. We screened the parameters which yielded more than 90% of total variance which the PC1 and PC2 accounted for to obtain accurate distribution of the data, as described in the manual of the PCA in Sigmaplot. When the three parameters of F/G, CIE a*, and CIE L* were subjected to PCA, the PC1 and PC2 accounted for 98.56% of the total variance which was the highest of all the combinations of three parameters (Table 3). The PC1 and PC2 which was obtained from the PCA of six parameters of F/G, CIE a*, CIE L*, ash content, free acidity, and F + G accounted for 89.38% of the total variance (Table 3). When the other six parameters which included either sucrose content or CIE b* were subjected to PCA, the PC1 and PC2 accounted for less than 85% of the total variance and the resultant PCA graph did not show good separation of the honey clusters (results not shown). Thus, the sucrose content and CIE b* were not used further for the PCA. The results in Table 3 indicated that F/G, CIE a*, CIE L*, ash content, free acidity, and F + G were in order of importance as parameters for accurate principal component analysis.
The PCA graph in Fig. 2 showed good separation of the four types of honeys, when the three parameters of F/G, CIE a*, and CIE L* shown in Table 3 were subjected to PCA. The PC1 had component loadings of 0.250, 0.989, and - 0.952 of F/G, CIE a*, and CIE L*, respectively, which indicated that the PC1 related weakly positively with F/G, strongly positively with redness, and strongly negatively with lightness. The PC2 had component loadings of 0.967, 0.0159, and 0.270 of F/G, CIE a*, and CIE L*, respectively, which indicated that the PC2 related strongly positively with F/G, very weakly positively with redness, and weakly positively with lightness.
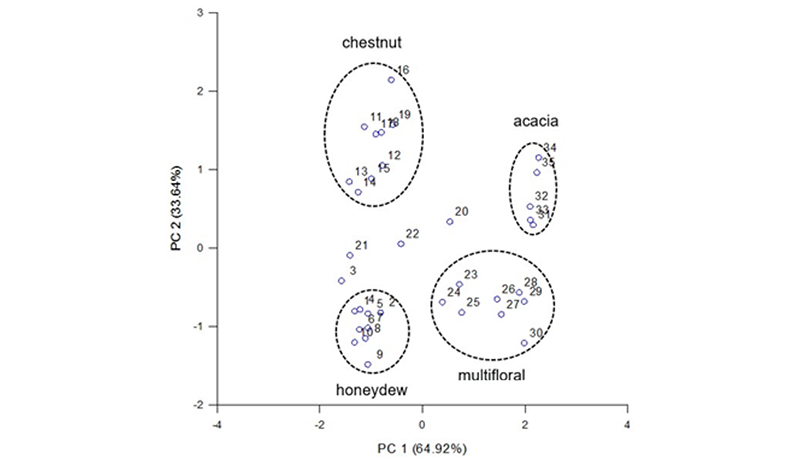
Fig. 2. Distribution of honeys in the principal component analysis graph which was drawn with the three parameters including fructose to glucose ratio, Commission Internationale de l'Eclairage (CIE, International Commission on Illumination) L* for the lightness from black (0) to white (100), a* from green (-) to red (+). PC1, principal component 1; PC2, principal component 2; 1 - 10, honeydew honey; 11 - 20, chestnut honey; 21 - 30, multifloral honey; 31 - 35, acacia honey.
Fig. 2 showed that the cluster of honeydew honeys 1 - 10 was located at the area with the negative ranges of both PC1 and PC2. The cluster of chestnut honeys 11 - 19 was located at the area with the negative and positive ranges of PC1 and PC2, respectively. The cluster of multifloral honeys 23 - 30 was located at the area with the positive and negative ranges of PC1 and PC2, respectively. The cluster of acacia honeys 31 - 35 was located at the area with the positive ranges of both PC1 and PC2. The honeydew honey 3, which had the highest F/G value of 1.13 and the highest CIE a* value of 10.42 of all the honeydew honeys, was located just above the honeydew honey cluster in Fig. 2, indicating that the honeydew honey 3 should have been mixed with chestnut honey. The chestnut honey 20, which had the lowest F/G value of 1.50, the lowest CIE a* value of 3.38, and the highest CIE L* value of 64.14 of all the chestnut honeys, was located at the region between the clusters of the chestnut and multifloral honeys, indicating that the chestnut honey 20 should have been mixed with multifloral honey. The multifloral honey 21, which had the lowest CIE L* value of 54.88 and the highest CIE a* value of 10.13 of all the multifloral honeys, was located at the region between the honeydew honey cluster and the chestnut honey cluster, indicating that the multifloral honey 21 was a mixture of honeydew and chestnut honeys. The multifloral honey 22, which had the second lowest CIE L* value of 59.71 and the second highest CIE a* of 6.64 of all the multifloral honeys, was located at the region between the chestnut honey cluster and the multifloral honey cluster, indicating that the multiflora honey 22 should have been mixed with chestnut honey. These results suggested that the four types of honeys might be differentiated efficiently by subjecting just three parameters of F/G, CIE a*, and CIE L* to the PCA. Even the multiple botanic origins of honeys located outside of the honey clusters might be determined using the PCA graph.
When either the four parameters of F/G, CIE a*, CIE L*, and ash content or the five parameters of F/G, CIE a*, CIE L*, ash content, and free acidity as shown in Table 3 was subjected to the PCA, the percentages of total variance which PC1 and PC2 accounted for were more than 90%. Thus, the obtained PCA graphs (results not shown) also showed good separation of the four types of honeys. The PCA graph in Fig. 3 also showed good separation of the four types of honeys, when the six parameters of F/G, CIE a*, CIE L*, ash content, free acidity, and F + G were subjected to the PCA. The PC1 had component loadings of - 0.373, 0.901, - 0.963, 0.932, 0.852, and - 0.812 of F/G, CIE a*, CIE L*, ash content, free acidity, and F + G, respectively, which indicated that the PC1 related strongly positively with redness, ash content, free acidity, weakly negatively with F/G, and strongly negatively with F + G. The PC2 had component loadings of 0.907, 0.402, - 0.139, 0.301, - 0.256, and 0.271 of F/G, CIE a*, CIE L*, ash content, free acidity, and F + G, respectively, which indicated that the PC2 related strongly positively with F/G, weakly positively with redness, ash content, and F + G, and weakly negatively with lightness and free acidity.
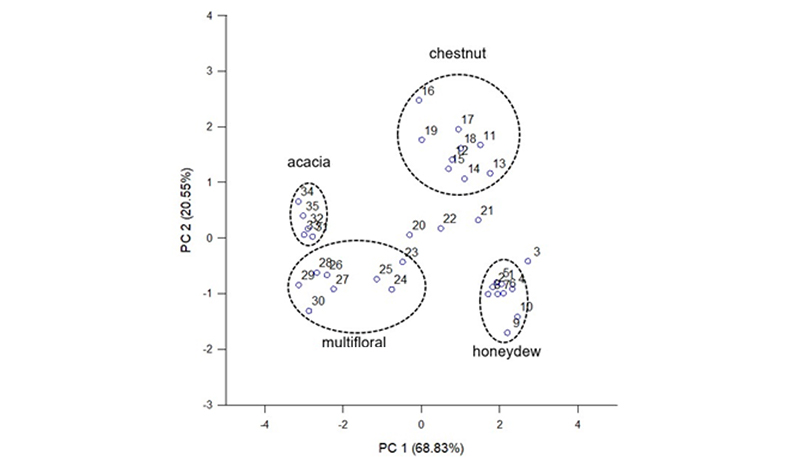
Fig. 3. Distribution of honeys in the principal component analysis graph which was drawn with the six parameters including fructose to glucose ratio, Commission Internationale de l'Eclairage (CIE, International Commission on Illumination). L* for the lightness from black (0) to white (100), a* from green (-) to red (+), ash content, free acidity, and fructose and glucose content. PC1, principal component 1; PC2, principal component 2; 1 - 10, honeydew honey; 11 - 20, chestnut honey; 21 - 30, multifloral honey; 31 - 35, acacia honey.
Fig. 3 showed that the clusters of honeydew honeys 1 - 10, chestnut honeys 11 - 19, multifloral honeys 23 - 30, acacia honeys 31 - 35 were located at the separate areas in the PCA graph. The relative locations of the honeydew honey 3, chestnut honey 20, multifloral honey 21, and multifloral honey 22 against honeys clusters were similar with those in Fig. 2. These results suggested that the four types of honeys might also be differentiated by subjecting the six parameters of F/G, CIE a*, CIE L*, ash content, free acidity, and F + G to the PCA.
The traditional method to determine botanical and geographic origin of honey is melissopalynology, the analysis of pollen in the honey. However, this method is time consuming, requires a specialist, and is unable to detect intentional pollen contamination. In general, in unifloral honeys, the pollen content should be at least 45% of the total pollen content, but this is not always the rule. For example, unifloral chestnut honey must contain more than 90% of chestnut pollen (Arvanitoyannis et al., 2005). Molan (1998) insisted that pollen analysis is valid only for the determination of geographic origin of honey, while it is less valid for determining the botanical origin. In some cases, most likely due to above-mentioned limitation of the pollen analysis, studies on the chemical composition of honey were performed without the pollen analysis. The botanical origin of honey is based on the claims of local beekeepers, when determination of honey origin is performed by sensory analysis or by considering the predominant flowers surrounding the hive (Kaškonienė and Venskutonis, 2010).
Principal component analysis of physicochemical data of honey has been used successfully to identify its plant origin (Maione et al., 2019). Sanz et al. (2005) analyzed thirty honey samples for pH, acidity, water, ash, net absorbance, total polyphenols, glucose, fructose, melezitiose, and erlose and the resulting data were used in PCA to characterize the samples in relation to their possible sources (nectar, honeydew, and mixture honeys). They did not classify the nectar honeys further into monofloral honey. Devillers et al. (2004) used twelve physicochemical parameters including moisture, HMF, conductivity, diastase activity, pH, free acidity, color, fructose, glucose, saccharose, erlose, raffinose, and melezitose in PCA to classify 469 honey samples into four clusters in the PCA of fir honey, chestnut honey, a mixture of heather, rape, and sunflower honeys, and a mixture of lavender and acacia honeys. Cotte et al. (2004) used seven sugar parameters including glucose, fructose, raffinose, trehalose, F/G, maltose/trehalose, and erlose/matulose in PCA to classify seven monofloral honeys into five clusters of fir honey, acacia honey, chestnut honey, a mixture of linden and lavender honeys, and a mixture of rape and sunflower honeys. They reported that the principal parameter distinguishing acacia and chestnut honeys from other five honeys was the F/G. The F/G of acacia and chestnut honeys were 1.7 and 1.5, respectively, and the F/G of five other honeys ranged from 1.0 - 1.3. Raffinose and trehalose contents were the principal parameters to distinguish fir honey from other six blossom honeys.
In this study, we also show that the F/G is one of the principal parameters in PCA for classification of honeydew, chestnut, multifloral, and acacia honeys. The PCA in this study requires just three physicochemical parameters for classification of the honeys. Additional advantage of the PCA in this study is that the physicochemical parameters, such as F/G and color, can be determined using the analytical instruments for composition criteria and quality control of honey.