Introduction
Bisphenol A (BPA) is one of the largest produced chemicals worldwide (Casey and Neidell, 2013; Corrales et al., 2015; Liguori et al., 2020), and is a widely used compound in the manufacturing industry, primarily used in the production of epoxy resins and polycarbonate plastics (Rahman et al., 2021). BPA is extensively applied in the manufacture of polycarbonate food containers and utensils, dental sealants, protective coatings, flame retardants, and water supply pipes (Wetheril et al., 2007).
BPA is identified as an environmentally persistent chemical and is reputed to be an endocrine disruptor due to its ubiquitous presence (Serra et al., 2019). Both animals and humans are exposed to BPA through food, beverages, inhalation and skin contact, resulting in the identification of BPA within biological fluids including saliva, sweat, urine, and blood (Dobrzyńska and Radzikowska, 2013). Concerns arise from its possible link with cardiovascular diseases, metabolic disorders, cancer, and sub-/infertility (Castellini et al., 2020). After several deviations, the European Food Safety Authority (EFSA) estimated ingestion of 4 µg·kg-1 body weight/daily, as the temporary tolerable daily intake (t-TDI) for BPA with the lowest-observed-adverse effect level for oral exposure (EFSA, 2015).
Recent reports have indicated the adverse effects of BPA on reproduction and development in animals and humans (Wetheril et al., 2007; Angle et al., 2013; Mínguez-Alarcón et al., 2016; Zhang et al., 2017). For example, BPA induces the proliferation of prostate cancer cells by combining with the mutant form of the androgen receptor found in human prostate tumors (Wetherill et al., 2002). Biochemical analysis revealed that BPA had a structural similarity to steroid hormones (especially estrogen), and displayed estrogen-like activity at concentrations below 1 ng·L-1 (Rykowska and Wasiak, 2006), thereby having the capability to alter almost every form of endocrine signal that is regulated by estrogens, androgens, and thyroid hormones. Thus, BPA was classified as a xenobiotic chemical that disturbs the hormonal balance in humans and other animals (Rykowska and Wasiak, 2006; Rochester, 2013; Serra et al., 2019).
In the last few decades, preclinical research has revealed the endocrine-disrupting effects of BPA on male reproductive functions. The possible mechanisms by which BPA interferes with spermatogenesis regulation, mainly via the hypothalamic-pituitary-gonadal axis, were also elucidated (Santiago et al., 2021). In rodent models, BPA was reported to affect sperm count, motility, DNA damage and litter size (Mínguez-Alarcón et al., 2016; Castellini et al., 2020). The current study was therefore undertaken to investigate the effects of BPA on reproductive function in young male mice, after the consumption of water containing BPA.
Materials and Methods
Animals and BPA consumption
Male the Institute of Cancer Research (ICR) mice (6 - 7 weeks) were purchased from Samtaco, Inc. (Osan, Korea), and rested for at least one week prior to use. All animals were provided a standard mice diet (Samtaco) and deionized/distilled water under conventional conditions (temperature: 20 - 22℃, humidity: 50 ± 5%, 12/12 hrs light/dark cycle). Bisphenol A (BPA; 4,4’-isopropylidenediphenol, 99% pure; #239658, Sigma-Aldrich Co., LLC., Seoul, Korea) was dissolved in 1 mL ethanol (99.9%), and diluted with deionized/distilled water. Mice were administered water with 50 mg BPA·kg-1 or without for 4 weeks. Daily water intake and body weight were measured every 7 days. All mouse experiments were performed according to the guidelines from the Animal Care and Use Committee protocol, and approved by the ACUC of Sunchon National University (SCNU IACUC-2021-08).
Unless otherwise noted, all other reagents used in this study were purchased from Sigma-Aldrich Chemical Co. (Seoul, Korea).
Breeding
After BPA intake for 4 weeks, male mice were randomly selected from each group for mating. A male mouse was housed in an individual cage with a virgin ICR female mouse, and was provided non-treated water (10 weeks age, 1 : 1 coupling) at night only (12 hrs dark), for 7 days. Pregnancy rate and litter size were investigated after breeding.
Examination of male reproductive organ and epididymal spermatozoa
Mice were euthanized by CO2 inhalation. Blood was aspirated using a 10 mL syringe, and centrifuged at 2,000 rpm for 10 min. The resultant serum was collected and stored at -20℃ until examination. Organs (liver, testes, and seminal vesicle) were excised and weighed on an electronic scale. The epididymis was dissected and washed thrice with phosphate-buffered saline (PBS). Using a surgical blade, the epididymis was minced in 2 mL PBS supplemented with 0.1% polyvinyl alcohol (PVA) (PBS-PVA; Yi et al., 2021), and incubated for 10 min at 37.5℃. Motility of the sperm released into PBS-PVA was estimated under a light microscope at 400 × magnification, and sperm concentration was estimated by a hemacytometer (Marienfeld-Superior, Germany).
Measurement of sperm proteasomal-proteolytic activity
The proteasomal-proteolytic activity of sperm has previously been described by Yi et al. (2012). Briefly, epididymal spermatozoa were loaded into a 96-well black plate at a sperm concentration of 1 × 105 spermatozoa/ml, and incubated at 37.5℃ with Z-LLE-AMC (a specific substrate for 20S chymotrypsin-like peptidyl-glutamylpeptide hydrolyzing [PGPH] activity not sensitive to MG132; final concentration 100 µM; Enzo Life Sciences, Plymouth, PA), Z-LLVY-AMC (a specific substrate for 20S proteasome and other chymotrypsin-like proteases, as well as calpains; final concentration 100 µM; Enzo), or ubiquitin-AMC (a specific substrate for ubiquitin-C-terminal hydrolase activity; final concentration 0.5 µM; Enzo). The fluorescence intensity was measured by a microplate reader (POLARstar OPTIMA, BMG Labtech, Ortenburg, Germany) at 380 nm excitation and 460 nm emission.
Serum testosterone assay
The testosterone competitive enzyme immunoassay kit was applied to determine the serum testosterone concentration, according to the protocol suggested by the manufacturer (Cayman Chemical, MI, USA). Briefly, the EIA kit was rinsed three times with washing buffer, and 50 μl testosterone standard and sample were added per well. Testosterone acetylcholinesterase tracer and testosterone antiserum (50 μL each) were incubated on a plate shaker for 2 hrs at room temperature (RT). The wells were emptied and rinsed five times with washing buffer. Ellman’s reagent (200 μL) was added to each well, and the plate was incubated for 1 hr and subsequently read at 405 nm on a microplate reader (BMG Labtech).
Hepatotoxicity assessment
Glutamate oxaloacetate transaminase (GOT) and glutamate pyruvate transaminase (GPT) were assayed by the Reitman-Frankel method (Reitman and Frankel, 1957). Serum levels were determined using the commercial detection kits, according to the manufacturer’s instructions (Asan Pharmaceutical, Seoul, Korea).
Histological assessment
Testis, epididymis, and seminal vesicles were harvested and fixed in 10% neutral-buffered formalin (Sigma). Fixed tissues were dehydrated through serial ethanol (50, 70, 80, 90, and 100%), cleared in xylene, embedded in paraffin, and finally sectioned as 5 μm thickness slices. Sections were subsequently deparaffinized in xylene and dehydrated through serial ethanol (100, 90, 80, and 70%), after which they were stained with hematoxylin for 5 min, washed in tap water, and counterstained with eosin for 15 min. Stained sections were dehydrated in graded ethanol (70, 80, 90, and 100%), cleared in xylene, and mounted with the mounting solution (Sigma) for further observation.
Statistical analysis
Values are exhibited as the mean ± standard error of the mean (SEM). Data analyses were conducted using unpaired-two-tailed t-test with GraphPad PRISMⓇ (GraphPad software, San Diego, CA, USA). Differences with p-values 0.05 at both tails are considered to be statistically significant.
Results and Discussion
Abnormal sperm production occurs in BPA-ingested mice
Male mice were administered water with or without BPA for 28 days. The body weight and the amount of water intake were examined every 7 days. During the experimental period, no significant difference was obtained in the body weight between groups (Table 1), as well as the amount of water consumed (Table 2). On the 28th day of water intake, the mice were sacrificed, and the weight of each organ was determined (Fig. 1). The weights of liver and testis (including epididymis and seminal vesicles) were significantly not different (Fig. 1A - 1C).
Table 1. Body weight of mice, ingesting water with or without bisphenol A (BPA).![]() |
y Mice drank water without (W/O) BPA or water containing BPA for 28 days. z Data are expressed as mean ± SEM. |
The motility and concentration of epididymal spermatozoa were measured after dissecting the epididymis (Fig. 2). Significantly higher sperm motility and concentration were obtained in mice consuming water without BPA, as compared to the BPA-ingested mice (p < 0.05; Fig. 2A and B). Sperm motility is the most important benchmark of sperm quality. During the reproduction process, motility is a critical factor that enables the sperm to move towards the oocyte to achieve fertilization (Suarez, 2008). In this study, exposure to BPA led to detrimental effects on sperm motility in male mice (Fig. 2A). Sperm motility is activated by adenosine triphosphate (ATP), which is generated from mitochondrial anaerobic glycolysis and respiration to supply adequate flagellar thrust to remain motile (Suarez, 2008; Tourmente et al., 2015). Previous studies have reported decreased sperm motility in the BPA-treated mice group, which may be due to supply loss of ATP (Richter et al., 2007; Dobrzyńska and Radzikowska, 2013; Cariati et al., 2019; Rahman et al., 2021; Santiago et al., 2021). It is assumed that BPA indirectly affects ATP production by abnormal mitochondrial formation during spermatogenesis.
In serum analysis, hepatotoxicity related to GOT and GPT levels was higher in BPA-ingesting mice than levels obtained in water-ingesting mice (p <0.05; Fig. 3A and 3B). According to Elhamalawy et al. (2018), these hepatotoxic alterations may be related to the induction of DNA damage by BPA in these tissues. Moreover, it has been reported that BPA causes oxidative damage in rat organs by generating the reactive oxygen species (ROS) (Chitra et al., 2003; Kabuto et al., 2004). BPA is also reported to alter the activities of ATPase in the liver and kidney of mice, thereby causing a reduction in the ATP production, resulting in necrosis (Sangai and Verma, 2012). The levels of GOT and GPT are the most sensitive indexes for liver injury. Even a slight change in the GOT and GPT activities in serum is indicative that liver function is damaged (Gu et al., 2021). In this study, we observed a high level of hepatotoxicity, which is expected to be the effect of BPA on the liver (Fig. 3A and 3B). The concentration of serum testosterone was also lower in BPA-ingested mice than mice without exposure to BPA (p < 0.05; Fig. 3C). Hematoxylin and eosin (H&E) staining of testicular sections revealed incomplete and irregular spermatogenesis in the testicular sections of BPA-ingested mice (Fig. 4A and 4B). Severe degenerative changes of round spermatids marked with pyknotic nuclei were also seen in the testis of BPA intake mice (Fig. 4B). No abnormal morphologies were observed in the epididymis (Fig. 4A’ and 4B’) and seminal vesicles (Fig. 4A” and 4B”) between groups. Spermatogenesis is a process that is coordinated by the hypothalamic-pituitary-testicular axis and the thyroid gland (Moriyama et al., 2002; Zoeller et al., 2005). Endocrine disruptors such as BPA cause triggering and dysfunction of this axis, which can lead to alteration of spermatogenesis. In recent studies, low doses of BPA were administered to mice, showing an impairment of spermatogenesis caused by the reduction of serum reproductive hormone levels in mice (Jin et al., 2013). Simultaneously, a reduction in the spermatozoa concentration was observed in the BPA administered mice (Cariati et al., 2019). In this study, as in previous studies, the BPA-treated mice showed low serum testosterone level (Fig. 3C), abnormal sperm production (Fig. 2A), and low concentration of spermatozoa (Fig. 2B).
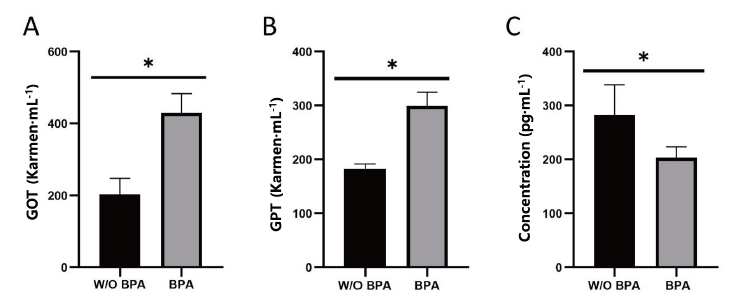
Fig. 3. Serum analysis of mice administered water with/without (W/O) bisphenol A (BPA). Hepatotoxicity level of glutamate oxaloacetate transaminase (GOT; A) and glutamate pyruvate transaminase (GPT; B), and concentration of serum testosterone were examined using serum. Values are expressed as the mean ± SEM. The superscript (*) denotes a significant difference at p <0.05.
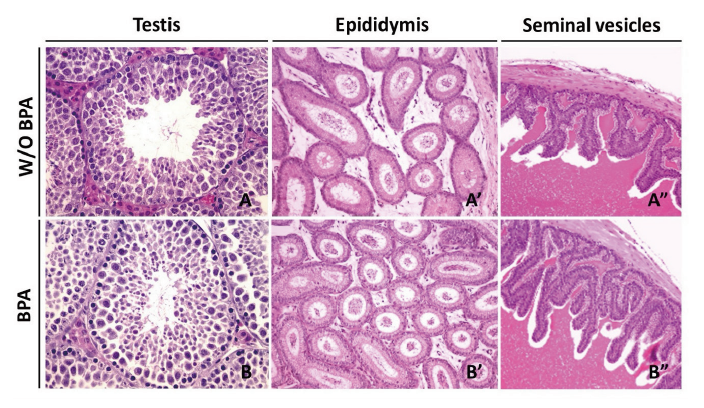
Fig. 4. Testicular section in mice consuming water with/without (W/O) bisphenol A (BPA). Testis (A and A’), epididymis (B and B’), and seminal vesicles (C and C’) were observed after hematoxylin and eosin (H and E) staining under × 200 (A - B and A’ - B’) and × 400 (A” and B”) mganifications of the light microscope.
Exposure to BPA affects the fertility competence
Previously, Sutovsky (Sutovsky, 2011) reported that the proteasome-proteolytic activity is implicated in sperm-oocyte interaction during mammalian fertilization. In the current study, no significant differences were observed in the fluorescent intensity for Z-LLE and LLVY between groups. Compared to controls without BPA, a significantly lower ubiquitin C-terminal hydrolase activity was observed in the BPA intake mice (p <0.05; Fig. 5). Evaluation of male fertility by breeding revealed a lower pregnancy rate in females mated with the BPA-ingested males; however, no significant difference was obtained in the litter size between the groups (Table 3). Consequently, the observed decrease in male fertility in the present study could be explained by the fact that BPA acts directly on the testes and influences the androgen biosynthesis pathway (Al-Hiyasat et al., 2002). However, direct adverse effects on the brain, hypothalamus or anterior pituitary gland will also indirectly affect the testes and possibly affect sexual activity. The significant decrease in the reproduction rate in females mated with males that ingested BPA may be attributed to an increase in preimplantation mortality of unhealthy fertilized ova due to alterations in sperm quality. In this study, ingestion of BPA caused a significant reduction in both epididymal and testicular sperm concentrations (Fig. 2). Such a reduction may be attributed to the direct effect of BPA on testicular Leydig and Sertoli cells, causing a decrease in testosterone production (Hughes et al., 2000). BPA has recently been shown to inhibit testicular Ca2+-ATPase, and it is postulated that these xenoestrogens disrupt testicular development by inhibiting the endoplasmic reticulum (ER) Ca2+ pumps, thus disturbing testicular Ca2+ homeostasis (Hughes et al., 2000). This aspect was not examined in the current study, but it is necessary to confirm ER stress of testis in follow-up studies.
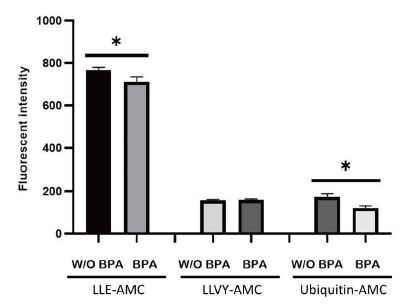
Fig. 5. Sperm proteasomal-proteolytic activity of sperm derived from mice ingesting water with/without (W/O) bisphenol A (BPA). Epididymal spermatozoa were incubated at 37.5℃ with Z-LLE-AMC (a specific substrate for 20S chymotrypsin-like peptidyl-glutamylpeptide hydrolyzing [PGPH] activity not sensitive to MG132), Z-LLVY-AMC (a specific substrate for 20S proteasome and other chymotrypsin-like proteases, as well as calpains), or ubiquitin-AMC (specific substrate for ubiquitin-C-terminal hydrolase activity). The fluorescence intensity (no unit) was measured using a microplate reader. Values are expressed as the mean ± SEM. The superscript (*) denotes a significant difference at p <0.05.
Conclusion
BPA is recognized as a potential endocrine disruptor that is known to affect fetal and adult life in animals and humans. BPA disturbs the cellular redox balance in testis and spermatozoa, resulting in altered testis development, architecture and function, impaired endocrine function, and abnormal semen parameters. Overall, it exerts adverse effects on sperm characteristics, including reduced sperm motility, concentration, and testosterone level. Therefore, careful application of BPA as a candidate for reproductive disturbance is needed, and further research on its control is required.
Acknowledgements
This work was supported by the National Research Foundation of Korea (NRF) grant funded by the Korean government (MSIT) (NRF-2013R1A6A3A04063769 and NRF-2020R1A2C1014007).
Authors Information
Young-Joo Yi, http://orcid.org/0000-0002-7167-5123
Malavige Romesha Chandanee, https://orcid.org/0000-0001-9236-7085
Dong-Won Seo, https://orcid.org/0000-0001-5299-5183
Jung-Min Heo, https://orcid.org/0000-0002-3693-1320
Min Cho, https://orcid.org/0000 0003 1606 6437
Sang-Myeong Lee, http://orcid.org/0000-0002-3624-3392