Introduction
Pig productivity depends on factors such as environmental stress and stocking density (Li et al., 2018). Stocking density increases frequency of contact, thereby increasing social tension and aggression (Hemsworth et al., 2013). A high stocking density impedes the movement of pigs because of limited space and has a negative effect on the health status of pigs (Cho et al., 2023). Thus, pigs under high stocking densities experience severe environmental, social, and psychological stresses. Lower densities allow more space for lying down and greater opportunities for pigs to regulate their body temperatures through behavioral adaptations (Li et al., 2020). Thus, it is common practice to employ nutritional strategies to minimize stocking density-associated decreases in productivity and increase adverse physiological and immune responses in growing pigs (Zhang et al., 2013; Cho et al., 2023).
Garcinia mangostana L., commonly known as mangosteen, is one of the best-tasting tropical fruits and is increasingly used as an active constituent in functional products (Wittenauer et al., 2012). The milky white portion of mangosteen is the edible portion, whereas the peel is dark red and approximately twice the mass of the edible portion (Fu et al., 2007). Fruit peels are a source of powerful natural antioxidants and are often the waste parts of various fruits from consumption and food industries (Suttirak and Manurakchinakorn, 2014). The use of food industry byproducts in livestock diets is becoming increasingly important owing to the increasing demand for cereals (Lei et al., 2018). Mangosteen peel was characterized both physically and chemically to reveal various xanthones, including alpha-mangostin, gamma-mangostin, garcinone C, garcinone D, garcinone E, gartanin, and smeathxanthone-A (Widowati et al., 2020). Alpha-mangostin, the first xanthone isolated from mangosteen peel, is a yellow coloring matter (Pedraza-Chaverri et al., 2008). It is also known that α-mangostin in mangosteen peel comprises approximately 69% of the total amount of xanthones (Wittenauer et al., 2012). Xanthone-active compounds can convert free radical compounds into more stable compounds and assist in the digestion process by improving the structure of the small intestine villi and suppressing the growth of bacterial pathogens inside the intestines (Widjastuti et al., 2021).
Mangosteen peel contains a variety of bioactive compounds with potential applications as functional food additives, such as phenolic acids, tannins, and anthocyanins other bioactive compounds (Choubey, 2018). Bioactive compounds isolated from mangosteen peels exhibit anti-inflammatory, antidiabetic, antioxidant, anticancer, antibacterial, antifungal, and antiparasitic activities (Shan et al., 2011; Suttirak and Manurakchinakorn, 2014; Ibrahim et al., 2016). Mangosteen peels have also been used as animal feed (Aizat et al., 2019). All in all, the mangosteen peel extract acts as a natural antioxidant and natural antibiotic which could be used to replace the anticoccidial drug additive in broiler feed (Sriboonyong et al., 2022). Several studies have shown that the addition of mangosteen peel has no adverse impact on the diet of dairy steers (Foiklang et al., 2016) and lactating cows (Polyorach et al., 2016) but improves the performance of broilers (Hidanah et al., 2017) and ducks (Kusmayadi et al., 2019). Mangosteen peel extract also shows anti-stress adaptogenic effects and improves the histopathological structure of some organs in mice (Jamaluddin et al., 2019; Rusman et al., 2021). However, there is no information available on the potential effects of mangosteen peel extract on growing pig diets.
Due to the antioxidant activity and immune enhancement function of dietary mangosteen peel extract (MPE), we hypothesized that increasing stocking density would result in increased levels of stress and, consequently, decreased growth performance of growing pigs, which could be partially or completely mitigated by dietary MPE supplementation. Therefore, this study aimed to determine the effects of stocking density and dietary MPE on growth performance, serum biochemistry, jejunal morphology, microbiome, and cytokine levels in growing pigs.
Materials and Methods
Animals, experimental design, and diets
The Institutional Animal Care and Use Committee of the Rural Development Administration approved this study (Approval No. NIAS-2022-2574), which proceeded at the swine facility of the National Institute of Animal Science of Korea. A total of 120 male growing pigs ([Landrace × Yorkshire] × Duroc) with an average initial body weight of 43.68 ± 0.48 kg were randomly assigned to 4 treatments with 6 replicates of 4 or 6 pigs in a completely randomized design and fed experimental diets with or without MPE (0 or 5 g/kg), thus leading to a 2 × 2 factorial design with stocking density. Growing pigs raised either at a stocking density of 0.55 m2/pig or 0.82 m2/pig were fed the diet for 6 weeks. All diets were formulated to meet or exceed the dietary requirements of the growing pigs (Table 1). MPE was purchased from MediBiolab (Korea). Feed and water were provided ad libitum throughout the experiment.
Sampling and measurements
Pigs were weighed individually on days 0 and 42, and feed intake was measured to calculate average daily gain (ADG), average daily feed intake (ADFI), and gain-to-feed ratio (G : F). The feed efficiency (G : F) was calculated as the ratio of body weight gain to feed intake.
On the last day, blood samples were collected from all pigs into serum-separating tubes and allowed to clot at room temperature for 1 hour. Serum was obtained after centrifugation at 1,800 × g for 15 min and frozen at -20℃ for analysis of serum characteristics. All pigs were then slaughtered and 5-cm long segments from the central part of the jejunum of each pig were washed and fixed in 10% buffered formalin for studying the intestinal morphology. Segments of the jejunum from all pigs were used to collect mucosal scrapings. The mucosal and spleen samples were frozen in liquid nitrogen and stored at -80℃ immediately after collection for further processing. For microbiome analysis, the fecal contents were isolated and stored at -80℃ until processing.
Serum biochemistry
Serum samples were analyzed for total protein (TP), globulin (GLOB), cholesterol (CHOL), phosphorus (PHOS), alanine aminotransferase (ALT), alkaline phosphatase (ALKP), gamma-glutamyl transferase (GGT), blood urea nitrogen (BUN) and creatinine (CREA) levels using a chemistry analyzer (Catalyst Dx, IDEXX Laboratories, Inc., USA).
Jejunal morphology
Jejunal morphology was determined as previously described (Shen et al., 2009). The fixed samples were dehydrated, embedded in paraffin, and cut into 5-μm-thick sections using a rotary microtome (Leica RM 2245, Leica Biosystems, Japan). The sections were fixed on glass slides and stained with hematoxylin and eosin. The stained slides were scanned and images were captured to measure the villus height (VH), crypt depth (CD), and VH : CD ratio of a minimum of 10 well-orientated villi in each jejunal segment using ImageJ (National Institutes of Health, USA).
Cytokine
Approximately 100 mg of mucosa and spleen were mixed in 1 mL 1X PBS (phosphate buffered saline) and homogenized (Digital Ultra-Turrax T25, IKA-Werke GmbH & CO., KG, Germany), respectively. The homogenate was then centrifuged at 5,000 × g for 5 min, and the aliquot of the supernatant was store at -20℃ until analysis. The TNF-α ELISA kit (PTA00, R&D Systems Inc., USA) and IL-10 ELISA kits (P1000, R&D Systems Inc., USA) was used to determine serum concentrations of TNF-α and IL-10. TNF-α ELISA kit (CSB-E16980p, Cusabio Inc., China) and IL-10 ELISA kits (CSB-E06779p, Cusabio Inc., China) were used to determine the mucosa and spleen concentrations of TNF-α and IL-10, respectively. The concentration of IL-2 (interleukin-2) in the serum, mucosa, and spleen were measured using an ELISA kit (CSB-E06783p; Cusabio Inc., China). The sIgA (secretory immunoglobulin A) concentration in the mucosa was measured using the ELISA kit (CSB-E12063p; Cusabio Inc., China). The protein concentration was determined using a bicinchoninic acid protein assay kit (Cat. 23225, Thermo Fisher Scientific Inc., USA).
16S rRNA Sequencing Analysis
Bacterial DNA was extracted from fecal content samples using the DNeasy PowerSoil Kit (Qiagen, Germany). The Illumina 16S Metagenomic Sequencing Library protocol was conducted then employed to amplify the V3 and V4 regions. The 2 ng of DNA was amplified with universal primers and Herculase II fusion DNA polymerase (Agilent Technologies, Inc., USA) under specific PCR conditions. After PCR, the products were purified with AMPure beads (Agencourt Bioscience, USA) and quantified using qPCR with KAPA Library Quantification kits (F. Hoffmann-La Roche Ltd., USA). The paired end sequencing (2 × 300 bp) was carried out using the Illumina MiSeq™ (Illumina Inc., USA) platform by Macrogen.
Following sequencing, Cutadapt (v3.2, https://cutadapt.readthedocs.io/) was used to remove adapter and primer sequences from the FASTQ files (Martin, 2011). For error correction, the DADA2 (v1.18.0, https://benjjneb.github.io/dada2/) package in R was applied (Callahan et al., 2016). This involved cutting the forward and reverse sequences to 250 bp and 200 bp, respectively, and excluding sequences with expected errors of 2 or higher. Corrected sequences were then assembled, generating amplicon sequence variants (ASVs) using the DADA2 consensus method. For comparative analysis of microbial compositions, QIIME (v1.9, https://qiime2.org/) was used to normalize the data (Caporaso et al., 2010). Each ASV sequence was subjected to BLAST+ (basic local alignment search tool, v2.9.0, National Center for Biotechnology Information, USA) against a reference database to assign taxonomy information (Camacho et al., 2009). Alpha diversity analyses (Chao 1, Shannon index, Shannon entropy) were conducted using QIIME to assess diversity and uniformity of fecal content samples. Beta diversity was visualized using principal coordinates analysis (PCA) with the help of STAMP software (v.2.1.3, https:// stamp-software.com/) (Parks et al., 2014).
Linear Discriminant Analysis Effect Size (LEfSe) Analysis
The linear discriminant analysis (LDA) effect size (LEfSe) analysis was carried out on the website (http://huttenhower. sph.harvard.edu/galaxy) (24th Jul. 2023) (Segata et al., 2011). The Kruskal–Wallis sum-rank test was performed to identify significant differences in the fecal microbiome between the two groups. Taxa with logarithmic LDA score values over 2.0 were selected for the histogram figure.
Statistical analysis
Data analysis was performed using the general linear model (GLM) procedure in SAS software (SAS Institute, USA), with individual pigs as the experimental unit. Tukey’s multiple comparison test was conducted to determine statistical differences among treatments (for interaction effects). Results are expressed as the mean ± standard error of the mean. The significance level was set at p < 0.05, and the tendency was set at p < 0.10.
Results
The effects of stocking density and dietary MPE supplementation on growth performance are presented in Table 2. No interaction effects on pig growth performance were observed between dietary MPE and stocking density. The FBW (final body weight) of growing pigs in the HD group was lower (p < 0.05) than that of pigs in the ND group. As stocking density increased, ADG and ADFI decreased (p < 0.05), and G : F was not affected. However, there were no significant differences in growth performance based on dietary MPE.
The effects of stocking density and dietary MPE on serum biochemical parameters are shown in Table 3. There was no interaction between stocking density and dietary MPE on the serum parameters in growing pigs.
Table 2
Effects of stocking density and dietary mangosteen peel extract (MPE) on growth performance in growing pigs.
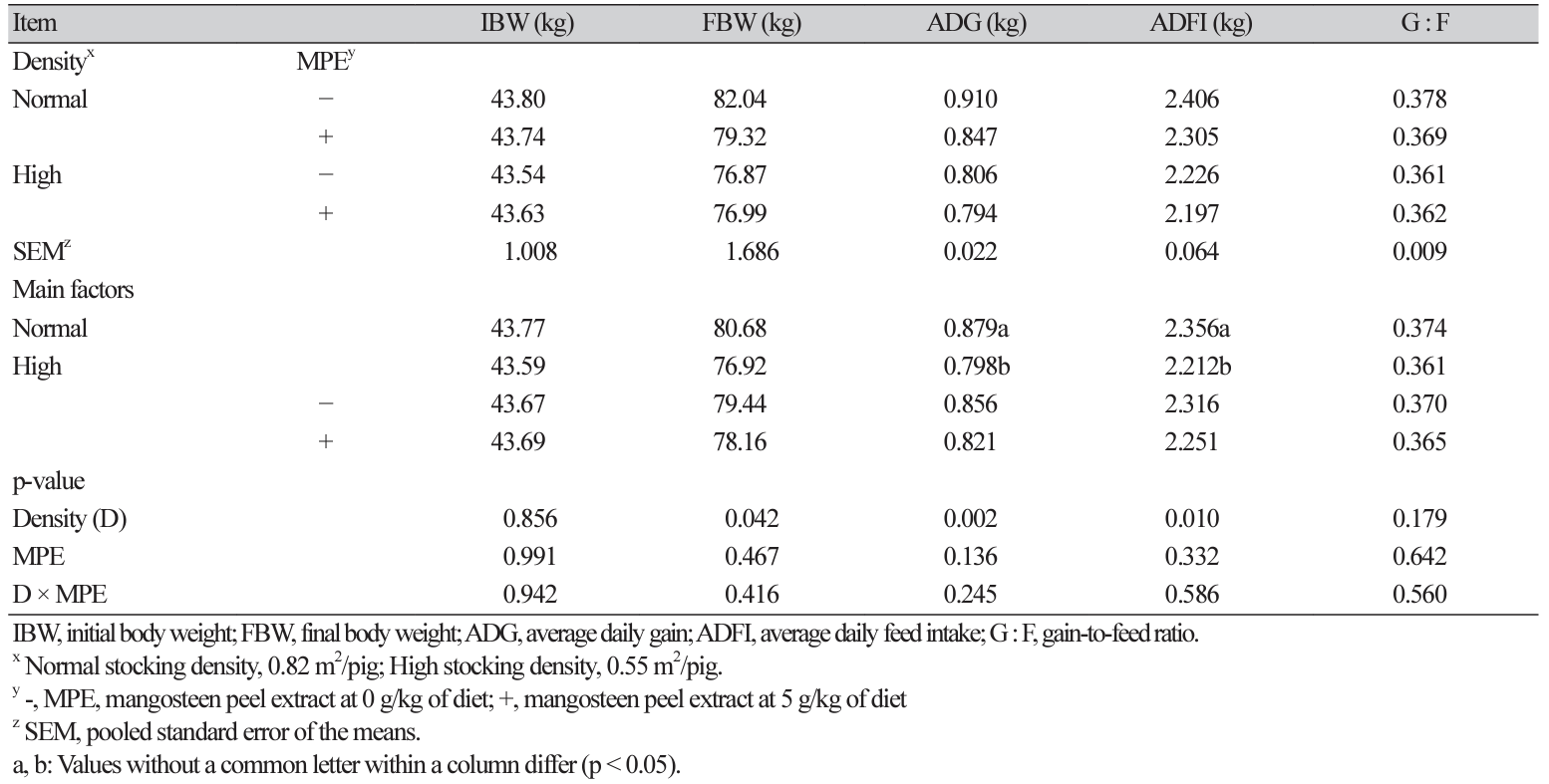
Table 3
Effects of stocking density and dietary mangosteen peel extract (MPE) on serum biochemistry parameters of growing pigs.
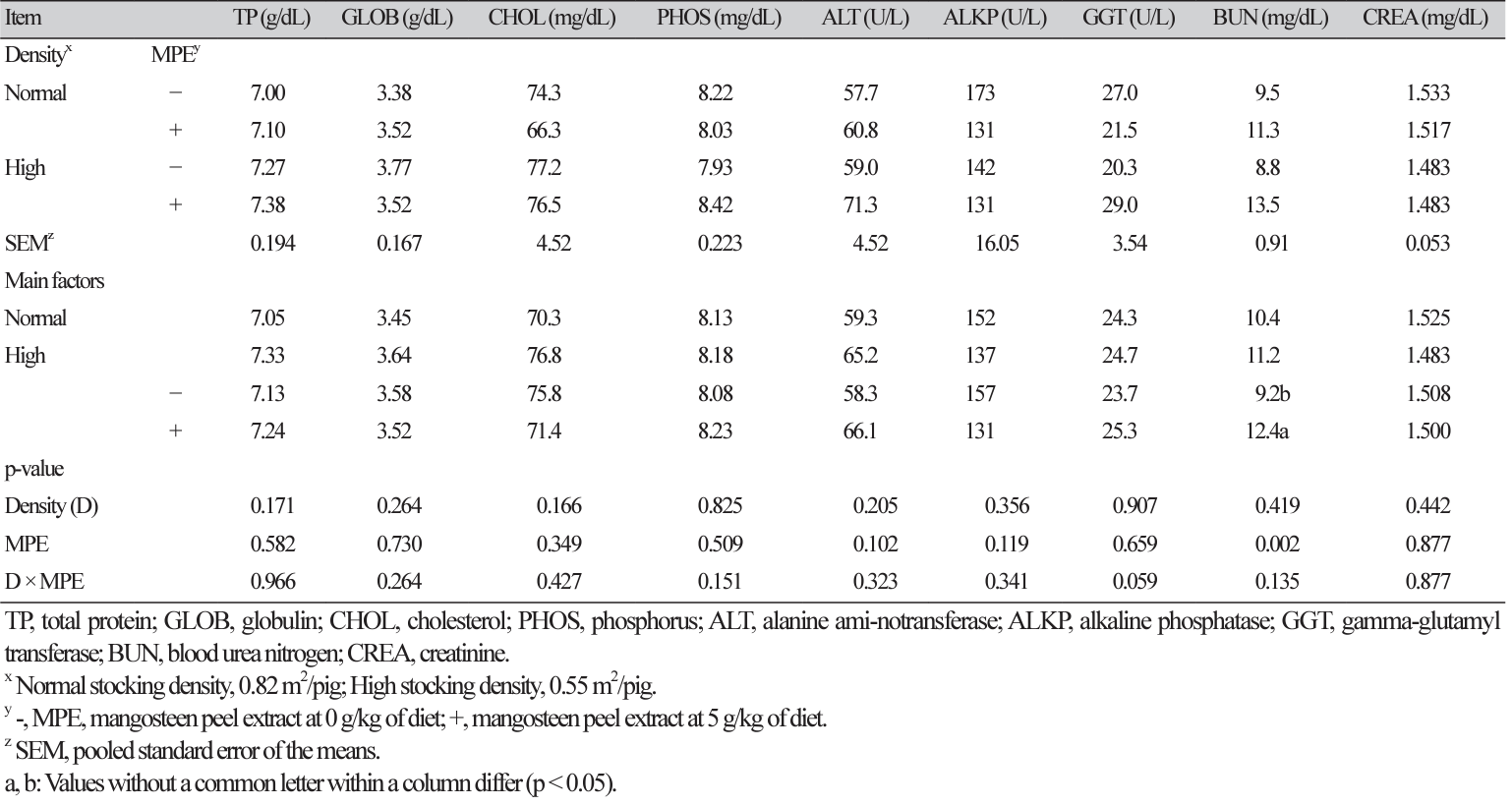
The effects of stocking density and dietary MPE on jejunal morphology are presented in Table 4. There was no effect of interaction between stocking density and dietary MPE on jejunal morphology in growing pigs. In addition, villus height, crypt depth, and the villus height-to-crypt depth ratio were not affected by dietary MPE or stocking density (p > 0.05).
The effects of stocking density and dietary MPE on cytokine levels in the serum, spleen, and mucosa are shown in Table 5 - 7. There was no interaction between stocking density and dietary MPE on the cytokine levels in growing pigs. Compared to the control group, pigs in the MPE group showed increased serum IL-10 levels (p < 0.05). No significant differences were noted for the content of TNF-α, IL-2, and IL-10 in spleen and mucosa among the groups (p > 0.05). The sIgA level of the mucosa increased in pigs raised on HD, whereas it was not affected by dietary MPE.
Table 4
Effects of stocking density and dietary mangosteen peel extract (MPE) on jejunum morphology in growing pigs.
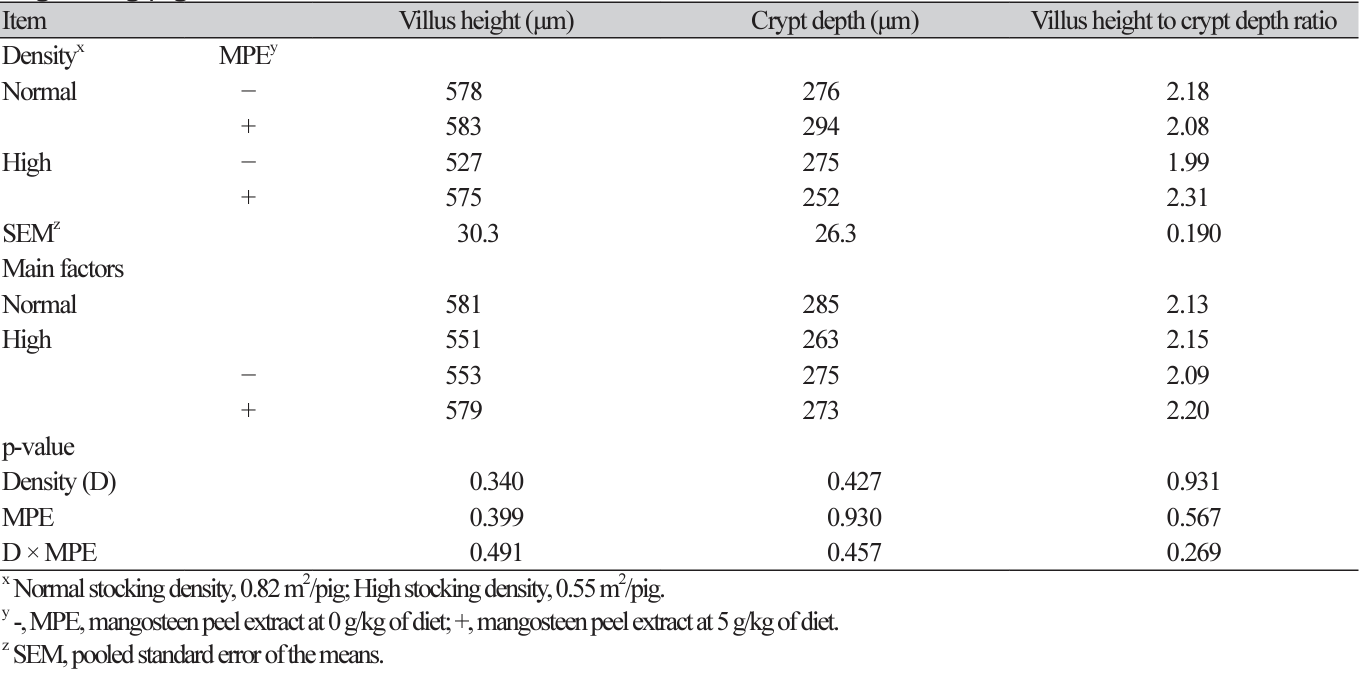
Table 5
Effects of stocking density and dietary mangosteen peel extract (MPE) on serum cytokines in growing pigs.
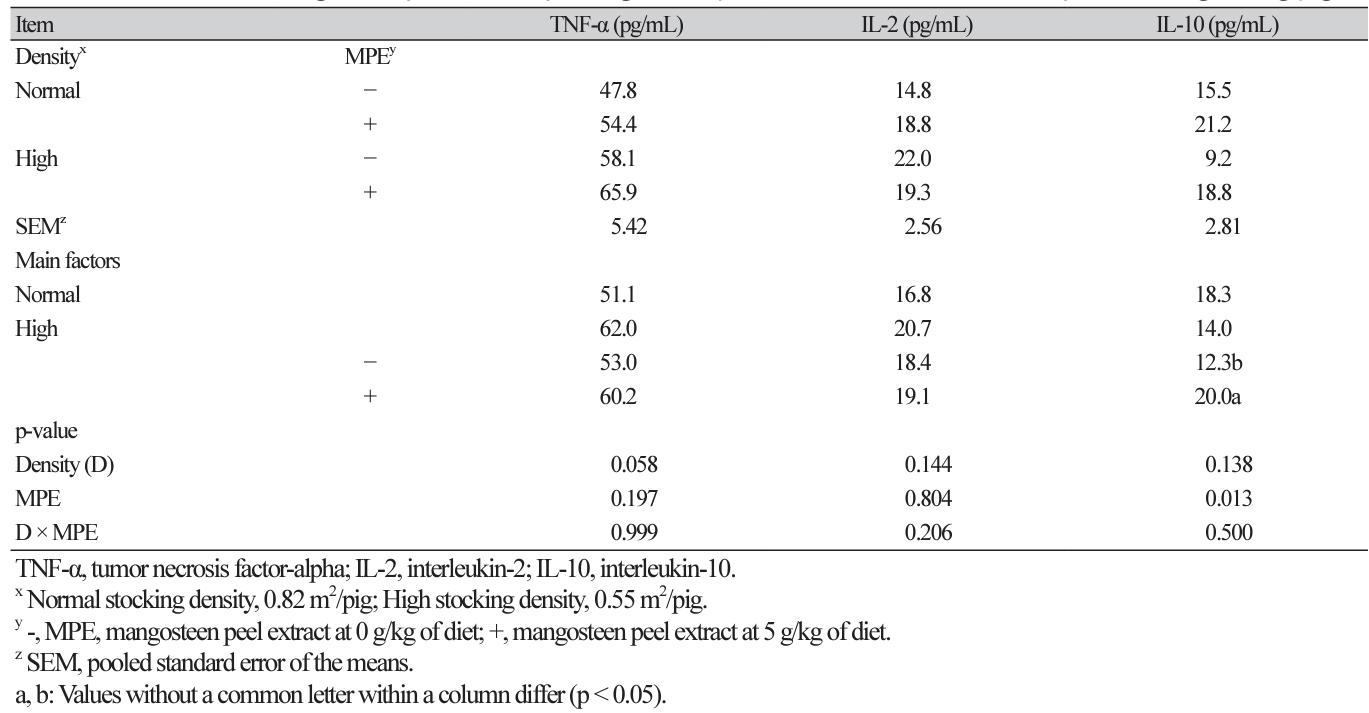
Table 6
Effects of stocking density and dietary mangosteen peel extract (MPE) on spleen cytokines in growing pigs.
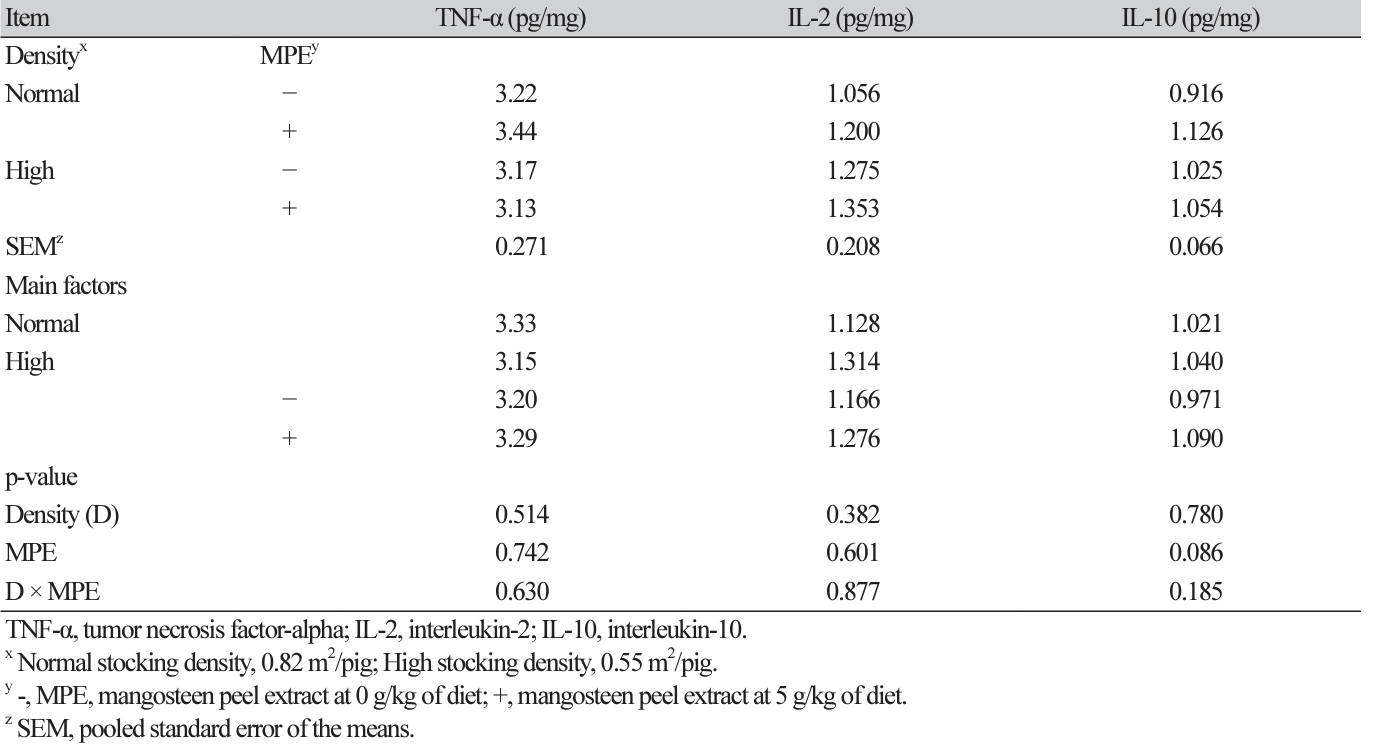
Table 7
Effects of stocking density and dietary mangosteen peel extract (MPE) on jejunum mucosa cytokines in growing pigs.
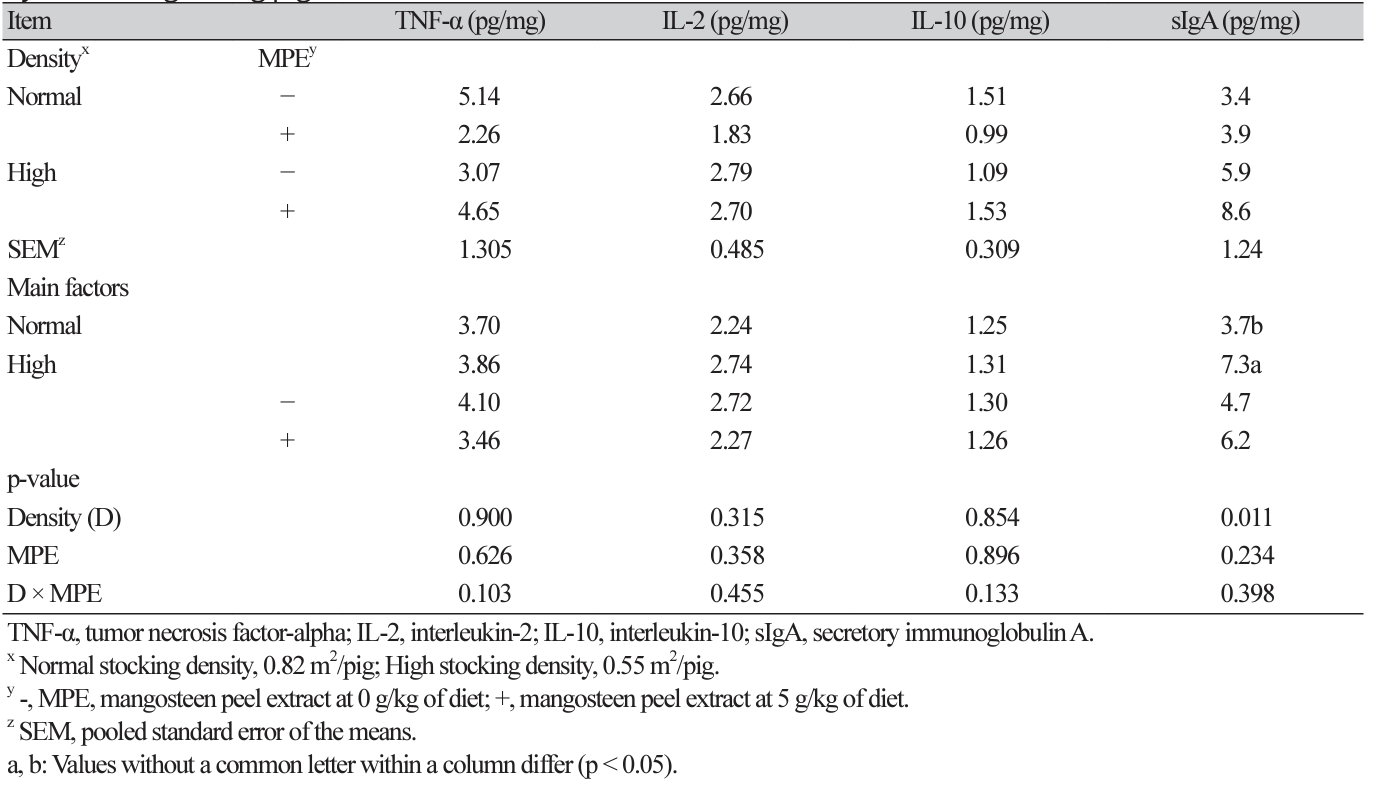
Metagenomic analysis were performed to examine the effects of MPE supplementation to gut microbiomes in pigs with normal and high density condition in pigs. The diversities of gut microbes in each group were assessed by alpha diversity analysis. In ASV, that indicating how many different microbes distributed, MPE effect was observed in normal density condition, but not high density condition. In Chao1 index, that indicating the difference of clusters of each sample group, MPE effect also was observed in normal density condition. MPE supplemented feed fed pigs had lower alpha and beta diversity in normal density condition but not in high density condition (Fig. 1A). There are no significant effects of density on ASV and Chao1 index. Simpson and Shannon_entropy were not differ among groups in this study (Fig. 1A). PcoA (principal coordinates analysis) analysis indicated that four groups were not clearly clustered. But, in general, MPE (diet) seems to have more significant effect on beta diversity compared with those of density effect.
The relative abundance of gut microbiome of pigs in four groups was examined at phylum, family, and genus levels. At the phylum level, firmicutes were dominant phylum (up to 70%) in gut microbes of pigs (Fig. 2A). Bacteroidets are secondary largest population of gut microbiome in pigs. At family level, each groups had a little different top three microbes in the gut (Fig. 2B). Prevotrellaceae (15.03%), oscillospiraceae (9.83%), and clostridiaceae (8.54%) were the top three families in ND-CON (control) group. In ND-MPE group, Prevotellaceae (16.19%), selenomoradaceae (9.14%), and eubacteriales (9.14%) are top three microbes. In HD-CON group, Streptococcaceae (13.52%), Clostridiaceae (11.26%), and Prevotellaceae (10.77%) are the case. Prevotellaceae (12.49%), Streptococcaceae (11.47%), and Christensenellaceae (10.54%) were top three microbes in HD-MPE group. And at genus level, also each groups had different top three gut microbiomes (Fig. 2C). Prevotella (13.60%), Clostridium (8.17%) and Barnesiella (7.55%) were top three genera in ND-CON group. Prevotella (15.24%), Eubacterium (7.98%), and Gemmiger (6.45%) were top three in ND-MPE group. In HD-CON group, Streptococcus (13.52%),
Fig. 1
Changes in gut microbial diversity in growing pigs according to diet and density. The alpha and beta diversity were analyzed in the four groups (ND CON, ND MPE, HD CON, HD MPE). (A) The alpha diversity index ASV (amplicon sequence variant), Chao1, Simpson, and Shannon entropy were analyzed. Boxplots represent ASV, Chao1, Simpson, and Shannon entropy. Statistical analysis was conducted by using two-way ANOVA. *p < 0.05, n = 6. (B) Principal coordinate analysis (PCA) was conducted for the beta diversity (n = 6). The axes (PC1, PC2) of the PCA explain 60.7% of the variance. ND, normal density; CON, control; MPE, mangosteen peel extract; HD, high density; D, density; PC, principal component.
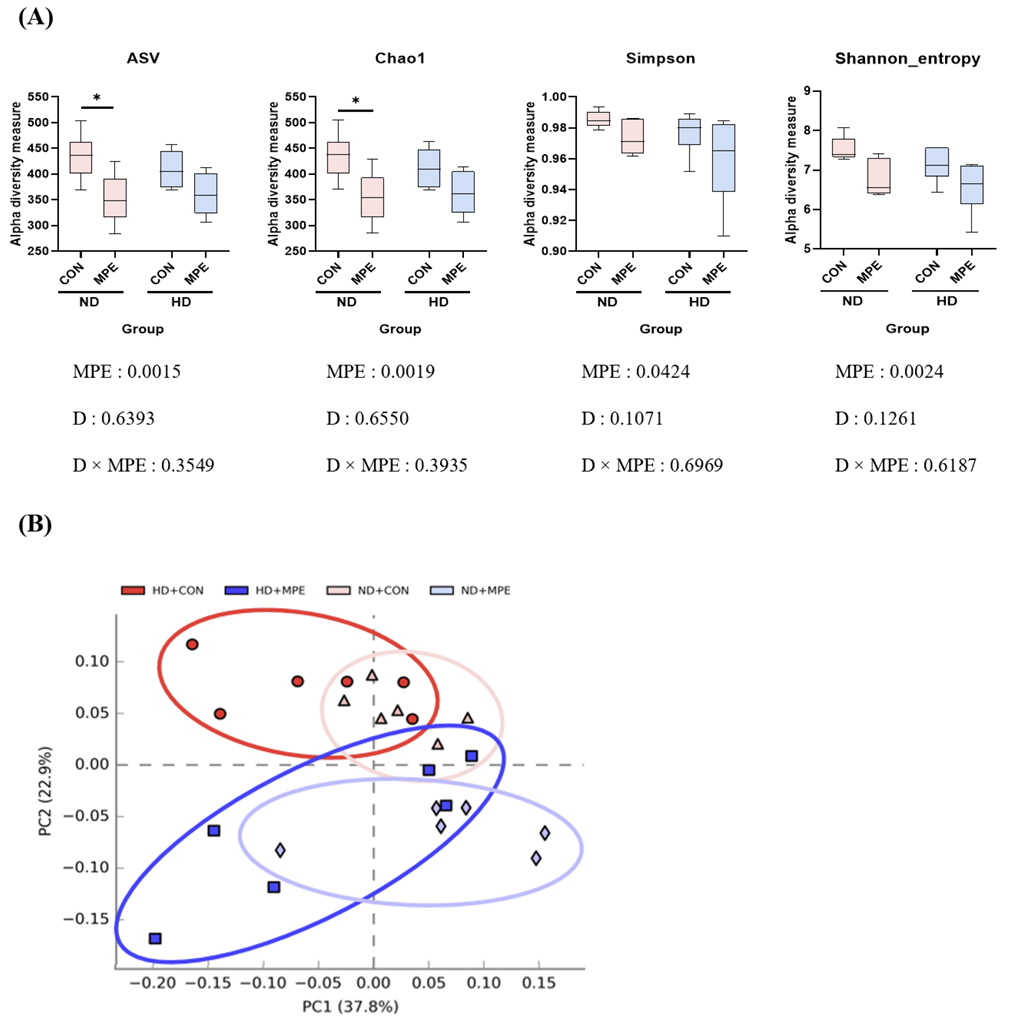
Fig. 2
Distribution of gut microbiota in growing pigs. Distribution of the predominant phyla, families, and genera in the microbiome of growing pigs. 16S rRNA sequencing was conducted to deter-mine the relative abundance at the phylum (A), family (B), and genus (C) level. Microorganisms that do not belong to the domain Bacteria have represented as “Others”. Microorganisms that were present in the sample at less than average 1% abundance were not included in the figure. ND, normal density; CON, control; MPE, mangosteen peel extract; HD, high density (continued).
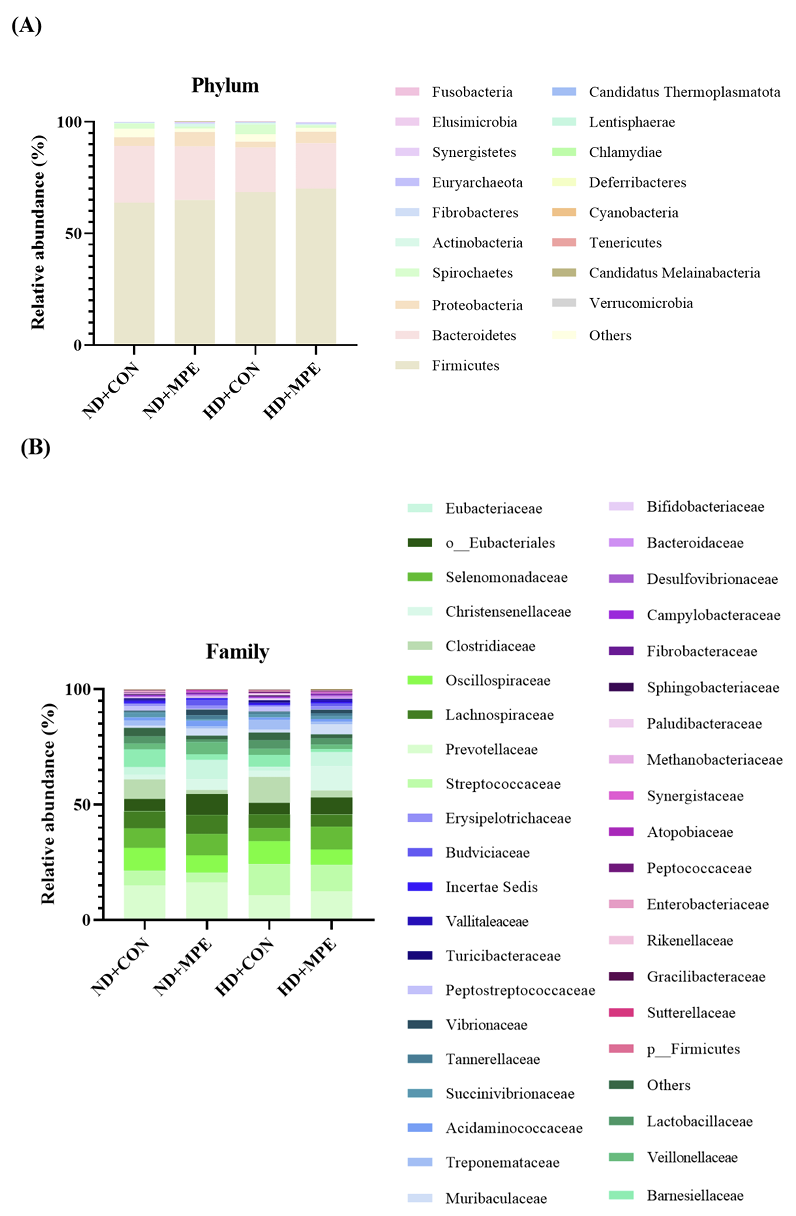
Fig. 2
Distribution of gut microbiota in growing pigs. Distribution of the predominant phyla, families, and genera in the microbiome of growing pigs. 16S rRNA sequencing was conducted to deter-mine the relative abundance at the phylum (A), family (B), and genus (C) level. Microorganisms that do not belong to the domain Bacteria have represented as “Others”. Microorganisms that were present in the sample at less than average 1% abundance were not included in the figure. ND, normal density; CON, control; MPE, mangosteen peel extract; HD, high density.
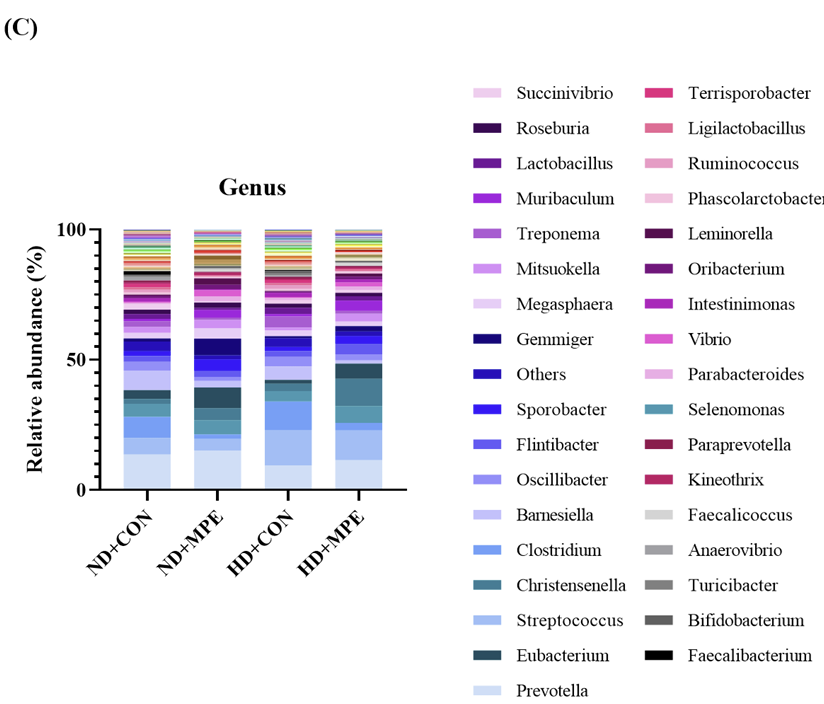
Clostridium (11.06%), and Prevotella (9.48%) are the case. And in HD-MPE group, Streptococcus (11.47%), Prevotella (11.43%), and Christensenella (10.54%) were the top three genera. Collectively, each group had a little different major microbial composition in the gut.
To identify significantly different taxa in abundance at the phylum to genus levels among four groups, LEfSe analysis were performed. Too see dietary effects, we examined significantly different taxa between ND-CON and ND-MPE. Gemmiger, Eubacterium, and Leminorella were top three elevated genera in ND-MPE group compared with that of ND-CON group (Fig. 3). Clostridium, Barnesiella, and Oscillibacter were top three decreased genera in ND-MPE group compared with that of ND-CON group (Fig. 3). To see stocking density effects, we examined significantly different taxa between ND-CON and HD-CON (Fig. 4). Mitsuokella, Butyricicoccus, and Rhodospirillum were top three decreased genera in HD-CON group compared with that of ND-CON group (Fig. 4A). Olivibacter, and Streptococcus were top two increased genera in HD-CON group compared with that of ND-CON group (Fig. 4A).
Fig. 3
LEfSe (linear discriminant analysis effect size) was used to identify significant microbial changes in growing pigs by MPE feeding. LEfSe analyses of gut microbiomes of ND MPE compared with ND CON. LEfSe identifies bacterial clades that are differentially abundant between ND MPE (green) and ND CON (red). The bar graph represents the LDA (linear discriminant analysis) scores for the ND CON and ND MPE groups. Clades in this graph were both statistically significant (p < 0.05) and had LDA score > 2.0, considered a significant effect size. (n = 6) ; p__is phylum, c__ class, o__order, f__family, and g__genus. ND, normal density; CON, control; MPE, mangosteen peel extract; HD, high density; LDA, linear discriminant analysis.
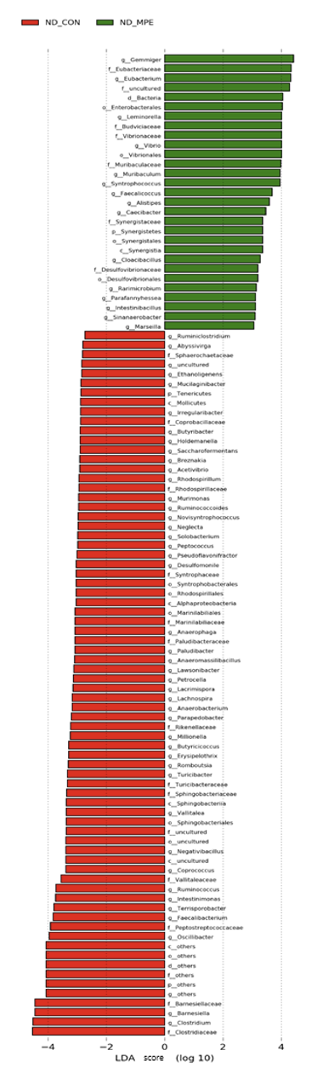
Fig. 4
LEfSe (linear discriminant analysis effect size) was used to identify significant microbial changes caused by high density and MPE treatment in growing pigs. LEfSe analyses of gut microbiomes of ND CON compared with HD CON. In the panel A, LEfSe identifies bacterial clades that are differ-entially abundant between ND CON (green) and HD CON (red). In the panel B, LEfSe identifies bacterial clades that are differentially abundant between ND MPE (green) and HD MPE (red). Clades in this graph were both statistically significant (p < 0.05) and had LDA score > 2.0, consid-ered a significant effect size. (n = 6); p__is phylum, c__ class, o__order, f__family, and g__genus. ND, normal density; CON, control; MPE, mangosteen peel extract; HD, high density; LDA, linear discriminant analysis.
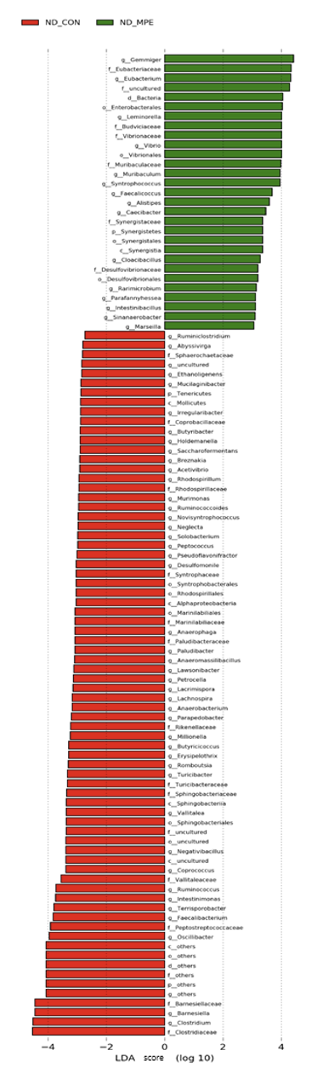
Significantly different taxa between ND-MPE and HD-MPE were presented in Fig. 4B. Syntrophococcus, Lachnospira, and Dialister were top three decreased genera in HD-MPE group compared with that of ND-MPE group. Streptococcus, Colidextribacter, and Anaerovorax were top three increased genera in HD-MPE group compared with that of ND-MPE group (Fig. 4B). Collectively, there are major microbial compositional changes by high stocking density stress depends on dietary treatment.
Discussion
Stocking density had a significant effect on pig growth performance. Previous studies on stocking densities have yielded similar results. Stocking densities less than 0.76 m2/pig result in a reduction in ADG and ADFI (Gonyou and Stricklin, 1998). Previous data indicated decreased ADG and ADFI with increasing stocking density (Wolter et al., 2002; White et al., 2008). White et al. (2008) reported that housing at 0.66 m2/pig resulted in pigs with a decrease of 17.0% in ADG and 10.7% in ADFI compared with pigs housed at 0.93 m2/pig. In our study, ADFI and ADG decreased with increasing stocking density, and it is generally thought that the reduction in body weight gain was a consequence of reduced feed intake. In addition, Yen and Pond (1987) reported no interaction effects were observed between dietary treatments and stocking density on pig growth performance.
Reports on the effects of dietary MPE on the growth performance of pigs at different stocking densities are scarce. Although MPE possesses antioxidant and immune properties, the use of dietary MPE in growing pigs has never been tested. Contrary to our expectations, dietary MPE did not affect the growth performance of growing pigs raised at high stocking densities. Similar to our findings, Pastsart and Pimpa (2022) found that supplementing MPE did not affect the growth performance of broiler chickens. Therefore, further studies are required to investigate the effects of MPE.
Serum biochemical parameters are useful indices for monitoring the health and physiological conditions of growing pigs and are affected by the growth stage, endocrine status, and dietary nutrient levels (Wang et al., 2009; Hong et al., 2020). It is clear from this study that the stocking density did not affect the serum biochemistry of growing pigs. In contrast to earlier studies (Li et al., 2020), we failed to observe stocking-density-induced increases in GGT and ALKP levels in serum samples. However, no clear explanation is apparent at this stage. Further research is required to elucidate this phenomenon.
Small intestinal morphological parameters, such as villus height, crypt depth, and their ratio, are important indicators of gut health (Han et al., 2013). Higher villus height resulted in an increased surface area, allowing for greater absorption of available nutrients and resulting in faster growth of broiler chicks (Sarikhan et al., 2010). In the present study, stocking density and dietary MPE did not affect jejunal morphology. We failed to observe a dietary MPE-induced increase in the villus height to crypt depth ratio in jejunal morphology. Thus, our study is consistent with the findings of Liu et al. (2013) reported that dietary plant extracts had no significant effects on intestinal morphology. Additionally, Li et al. (2020) reported no significant differences in the villus height, crypt depth, or height-to-crypt depth ratio of the jejunum among different stocking density groups in growing pigs.
Cytokines play a critical role in the immune response, inflammatory response, and impairment of intestinal barrier function (Liu, 2015). In this study, dietary MPE increased the serum levels of the anti-inflammatory cytokine IL-10. It is likely that dietary MPE enhances intestinal immune function by regulating cytokine production. However, it is clear from this study that stocking density did not affect TNF-α, IL-2, and IL-10 levels in the serum, spleen, and mucosa, and did not interact with dietary MPE. In contrast, the levels of IL-2 and IL-10 in the spleen of growing pigs in the high stocking density group were higher than those in the normal density group (Li et al., 2020).
In the present study, although the stocking density did not affect the TNF-α, IL-2, and IL-10, it affected the sIgA in jejunum mucosa. Similarly, Li et al. (2020) reported that sIgA levels in the jejunal mucosa of growing pigs in a high stocking density group were higher than those in a normal density group. Mucosal sIgA protects the mucosal surface and is a critical component of the intestinal immune barrier (Wells et al., 2017). The sIgA response to an enteric pathogen is the first immunological reaction to infection (Crivelli et al., 2001). Therefore, the promotion of sIgA secretion stimulates intestinal mucosal immune protection mechanisms (Li et al., 2020).
The gut microbiome is closely related to health status of animals. This is a state that has been found to be applied to various livestock in previous studies, and pigs are also applicable (Patil et al., 2020). The composition of the pig's microbiome changes by various factors including diet and stress. In this study, we examined the changes of gut microbiome by MPE supplementation and high stocking density stress.
In the alpha diversity, number of ASV and Chao1 index were significantly decreased in ND-MPE group in the comparison of ND-CON (Fig. 1A). In addition, the beta diversity was presented through PCA plots, it was shown that they grouped by dietary MPE feeding rather than density stress conditions (Fig. 1B). These results indicated that MPE significantly affected to gut microbiome in pigs, especially in normal density condition. In general, reduced gut microbial diversity considered as dysbiosis condition. This may be explained by antibacterial function of MPE (Soetikno et al., 2016) arising from the abundant presence of xanthones such as α-mangostin and β-mangostin in the mangosteen peel (Indrianingsih et al., 2020; Budiati et al., 2022). The fact that whether MPE had beneficial or harmful effects on gut microbiome need to be further studied in the future. Various taxa changes caused by dietary MPE were presented through LEfSe analysis. The top 3 increased genera by ND-MPE treatment were Gemmiger, Eubacterium, and Leminorella. There is not much information about functional role of these microbes in pigs. In probiotics or synbiotics addition to sows’ feed study for offspring pigs, Gemmiger showed correlation with short-chain fatty acids (SCFAs), such as acetate, propionate, and butyrate (Zhu et al., 2022). SCFAs are considered as beneficial functional microbial metabolites in terms of productivity and health. Eubacterium spp. have been described a group of beneficial bacteria (Mukherjee et al., 2020). Recently, gut microbiologists suggested that specific strains of butyrate-producing microbe belonging to the genera Eubacterium and they may ultimately be considered as beneficial bacteria, like strains of Lactobacillus and Bifidobacterium (Salminen et al., 2021). In addition, Eubacterium spp. contribute to gut and hepatic health through modulation of bile acid metabolism. E. hallii possess the enzyme bile salt hydrolase can hydrolyze the C-24 N-acyl amide bond in conjugated bile acids to release glycine and taurine moieties which are good for gut health (Udayappan et al., 2016). And the top 3 decreased genera were Clostridium, Barnesiella, and Oscillibacter. Clostridium was decreased by dietary MPE. Clostridium spp., as a predominant cluster of commensal bacteria in gut, exert many effects on gut homeostasis (Guo et al., 2020). Clostridium have been reported to related to inflammation and allergic diseases development. Some of clostridium species are considered as pathogen. For example, C. difficile damages digestive system, especially colon, via its toxin production (Songer, 2010). C. difficile infection often occurs after antibiotics therapy. When part of commensal bacteria are eliminates, C. difficile rapidly grow owing to imbalance between gut microbiome and gut immune system. However, some Clostridium spp. are considered beneficial microbe, because they can ferment a variety of nutrients, like carbohydrate, protein, organic acids (Guo et al., 2020). C. butyricum LY33 that isolated from piglet intestinal contents have positive effects on gut metabolism of weaned piglets (Hu et al., 2019). It revealed that this microbe improved gut health through efficient utilization of lactates and butyrate conversion. In addition, it had an inhibitory effect on pathogen such as salmonella. In our study, Clostridium genus was reduced by dietary MPE, but as mentioned previously, they can be considered either as beneficial or harmful microbes depending on the species levels. Therefore, it is hard to determine whether decreased clostridium by MPE is beneficial or harmful for pigs in this study.
In livestock industry, pigs are under a lot of stresses in the breeding environment. Among them, overcrowding is one of the main stress factors to be considered. Due to the nature of the livestock industry, which overcrowding that breeding a large number in a small area are easily occur. In general, over density breeding known to reduce productivity by pigs. In this study, we examined change of gut microbiome by high density breeding stress (Fig. 4A). Mitsuokella, Butyricicoccus, and Rhodospirillum genera were decreased by high density breeding condition. However, Olivibacter, and Streptococcus genera were increased by high density breeding condition. Mitsuokella jalaludinii, which is isolated from pigs or piglets was reported that it can inhibit of salmonella growth, and featured the production of carboxylic acids such as lactate, succinate, or acetate (Levine et al., 2012). In addition, it has been previously shown to produce phytase which can provide beneficial gains in feed efficiency (Tang et al., 2021). In this study, another decreased genus by high density stress condition is Butyricicoccus. In the metagenomic analysis of pig fecal microbiome, Butyricicoccus was related in feed efficiency (Quan et al., 2020). From the weaning piglets to 140-days old, pigs grew, and among them, the groups were divided into low feed efficiency and high feed efficiency. Compared with low feed efficiency pig group, 17 genera that were potentially associated with feed efficiency were enriched, including butyricicoccus. This may explain decreased productivity of pigs in high density breeding condition. In general, high density stress reduces beneficial microbes such as Mitsuokella and Butyricicoccus in our study.
In this study, we also examined the effect of MPE supplementation on high density induced gut microbiome alteration. We compared the composition of gut microbiome between ND-MPE and HD-MPE to determine whether MPE supplementation affect altered gut microbiome by high density breeding stress. With MPE dietary treatment, gut microbial taxa such as Mitsuokella and Butyricicoccus that altered by high density condition in the context of non-MPE supplemented diet were not differ between normal and high density condition. These suggests MPE treatment have certain effects on gut microbiome shift by high density breeding stress in pigs.
Streptococcus is increased genera in MPE fed pigs by treatment HD stress. Streptococcus is one of the microbial groups present in the intestines of pigs. Among them, Streptococcus suis is one of the globally significant swine pathogens, causing septic diseases leading to septicemia and acute death, among others. Since Streptococcus suis causes common diseases in both pigs and humans. As a result, the management of pig infections is highly regarded for the prevention and control of potential human infections (Zimmerman et al., 2019). Based on this, providing MPE treatment can be considered beneficial for reducing Streptococcus infections, contributing to the management of infections in both pigs and humans. Syntrophococcus, Lachnospira, and Dialister are decreased in MPE fed pigs by treatment HD stress. Syntrophococcus, a decreased microbe under HD stress conditions, belongs to the Lachnospiraceae family. This family is known for its beneficial microbes, which are active members of the gut environment, aiding in the decomposition of indigestible plant components like cellulose into SCFAs (short-chain fatty acids) in ruminants (Biddle et al., 2013). This decomposition enables the host to utilize plant components that were previously unavailable as an energy source. This concept can also be applicable to pigs, not just ruminants. Previous research has shown that in pigs with higher feed efficiency, there was a higher proportion of Syntrophococcus in the gut. This suggests that Syntrophococcus may contribute to improving gut health and increasing feed efficiency in pigs by assisting in digestion and converting indigestible components into SCFAs, similar to its role in ruminants (Aliakbari et al., 2021).
Lachnospira is also a member of the Lachnospiraceae family. In addition, there is a study showing that Lachnospira was more abundant in the group of pigs that did not experience post-weaning diarrhea in susceptible and healthy pig groups (Dou et al., 2017). This study suggests that a higher abundance of Lachnospiraceae not only reduces post-weaning diarrhea but also enhances energy harvesting and protects pigs from pathogen infections.
Dialister is also known as a SCFAs-producing microbe, and there is a study that shows an increase in Dialister in piglets vaccinated with the same vaccine as mentioned earlier (Luise et al., 2020). As mentioned before, the increase in SCFAsproducing microbes can be considered beneficial for improving the host's gut environment.
Based on this, providing MPE feed can be considered to have contributed to the increase in SCFAs-producing microbes, including those from the Lachnospiraceae family (such as Syntrophococcus, Lachnospira) and Dialister, leading to improved feed efficiency and promoting gut health in pigs.
However, these microbial changes are not sufficient to improve HD-induced stress response as reduced productivity was not return to normal level.
Conclusion
Collective, MPE supplementation had significant effects on gut microbial diversity and composition of gut microbes in growing pigs. Increased Lachnospiraceae family and Dialister can promote gut health in growing pigs. However, these changes could not lead to improve productivity in the context of HD stocking stress condition. This suggests that MPE have regulatory potential for gut microbiome, but further studies are necessary to understand functional role or effect of altered microbiome on pigs.
Ethical Approval
This study was approved by the Institutional Animal Care and Use Committee of the Rural Development Administration (approval no. NIAS-2022-2574), conducted at the swine facility of the National Institute of Animal Science of Korea.
Acknowledgements
This work was supported by “The Cooperative Research Program for Agriculture, Science and Technology Development (Project No. RS-2021-RD010035) of the Rural Development Administration, Republic of Korea.
Authors Information
Minji Kim, https://orcid.org/0000-0003-2106-1921
Jin Young Jeong, https://orcid.org/0000-0002-8670-7036
Nam-Geon Park, https://orcid.org/0000-0001-6241-2850
Eunju Kim, https://orcid.org/0000-0003-4040-0474
Sang Seok Joo, https://orcid.org/0000-0001-8909-1102
Moongyeong Jung, https://orcid.org/0009-0003-9194-2887
Myunghoo Kim, https://orcid.org/0000-0002-8444-6952
Yoo-Bhin Kim, https://orcid.org/0000-0001-8417-6967